Using Fluorescent Recombinant Proteins to Validate CAR-T Cell Therapy
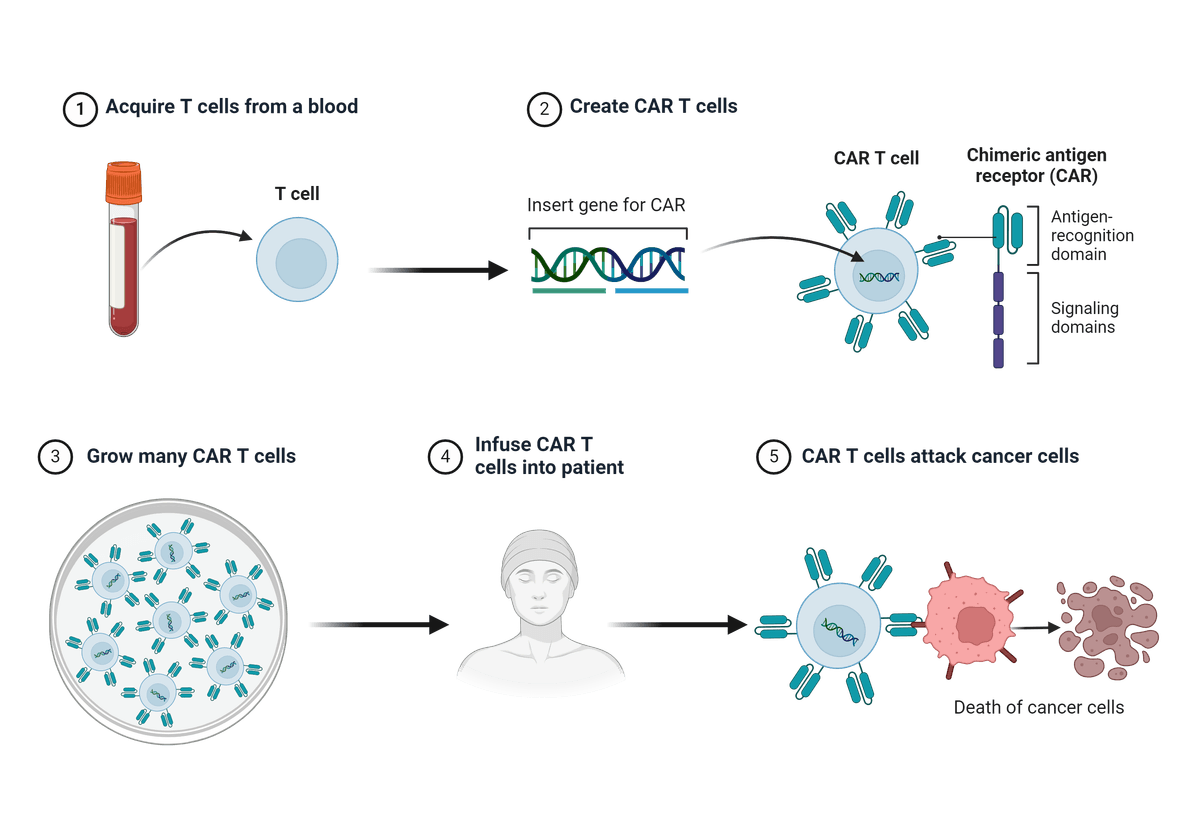
Illustrated step-wise overview of Chimeric Antigen Receptor (CAR) T cell generation and cancer therapy. Figure created with BioRender.com.
However, not all patients will respond favorably to CAR T-cell therapy, and the treatment of solid tumors in particular still poses many challenges. Therefore, the validation of the efficacy of a CAR T-cell therapy is particularly important to provide important patient-specific data on the possible success or failure of a given treatment.
Ways to Detect, Quantify, and Visualize CAR
There are a number of ways that can be used to validate a CAR T-cell therapy, at various levels of scale. At the small scale, real-time quantitative PCR (qPCR) can measure the vector copy number of your replicated CARs, integration site analysis may help determine local sites of mutagenesis, and RNA-seq can help determine the abundance of CAR mRNA. For use in proteomics, flow cytometry and Western blot can help quantify the CAR population. Bigger-picture CAR visualization experiments have become increasingly important in recent years, with much research focused on fluorescence microscopy. bioluminescence imaging, positron emission tomography (PET) scanning, and two-photon microscopy to determine CAR-T cell movement within organs and tissues. Indeed, there is still much to learn about the molecular mechanisms behind CAR, and further insight may help inform genetic engineering efforts to improve CAR T-cell therapies for the future.
A popular method of evaluating CAR expression is through flow cytometry, typically through the use of a target antigen because they offer high specificity with little background. The use of fluorescent-labeled target antibodies as detection probes, like PE, FITC, or APC, is a highly efficient technique, with a great level of detection.
Application Note: |
Alternatively, biotin-streptavidin detection can be used which offers a uniquely high affinity and specificity for CAR detection. Unconjugated proteins can also be used as an indirect detection method; target antigens carry a specific tag that becomes detectable using a secondary antibody labeled with a fluorophore. If using this method for CAR detection, it is important to remember that the secondary antibody may cause non-specific labeling which may provide higher levels of background noise upon analysis.
Benefits of Fluorescent Recombinant Proteins as Validation Systems
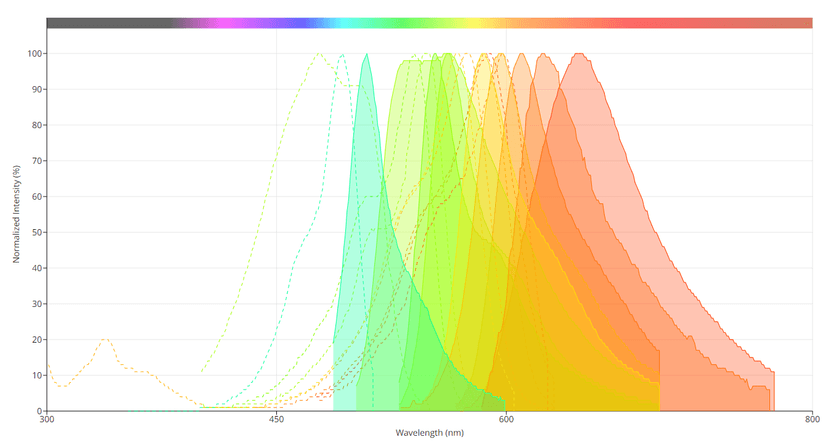
Spectral profiles of several of the 'mFruits' fluorescent proteins. Image from the AAT Bioquest Fluorescence Spectrum Viewer.
Fluorescent recombinant protein technologies coupled with flow cytometry analysis have also proven useful in profiling protein expression among different sub-cellular populations. For these reasons, the use of fluorescent recombinant proteins holds much potential in validating CAR T-cell therapies.
Using Fluorescent Proteins to Advance CAR T-Cell Therapy
The use of fluorescent proteins to advance understanding of CAR is growing fast, and offers great potential to optimize CAR T-cell-based medicine. In one study, Fluorine-19 (19F) magnetic resonance imaging (MRI) was used to noninvasively detect 19F perfluorocarbon labeled cells (PFC+) CAR-T cells in mouse models of leukemia (Dubois, 2021). The study demonstrated that a clinical field strength MRI could be used to visualize labeled cells for up to 7 days post-injection, and that PFC labeling did not significantly affect in vivo CAR T-cell cytotoxicity. This research demonstrated the potential for the use of such imaging tools in tracking emerging CAR T-cell therapies in preclinical models and, eventually, patients.
Another study took a multiplexed approach to rapidly identify the clinical potential of different CAR designs using GFP as a marker (Goodman, 2022). This high-throughput approach screened and tested forty different CAR structures that had various signaling domains derived from a range of immune cell lineages. CAR T-cells were labeled with a 2A-GFP marker, and then evaluated in a pooled assay for their ability to stimulate critical T-cell effector functions. FlowSeq, a pooled flow cytometry-based method, was used to quantitatively measure the fluorescent readout across the cell population at specific time points. Specifically, FlowSeq measured different markers of T-cell function, including activation, cytokine production, and proliferation between different cell types (CD4, and CD8 T-cells), at both early and late stages of antigen stimulation and expansion. Ultimately this research may help advance CAR engineering efforts and enhance their ability to target and eliminate specific cancers.
A different study aimed to maximize the anti-tumor efficacy of a novel CAR targeting glypican-2 (GPC2) prior to introducing these cells into patients with newly diagnosed high-risk neuroblastoma (Sun, 2023). The team found that among patients who went into remission after the initial therapy, over 50% experienced relapses due to minimal residual disease. In the research, first stable luciferase (ffLUC)-GFP-expressing cells were generated, and then the CAR T-cells and ffLUC-GFP-expressing NB tumor cells were co-cultured at varying effector-to-tumor ratios. Each day, a specified amount of tumor cells was added to the population to rechallenge the function of the CAR T-cells. To quantify the reaction, the lysis of the tumor cells was measured via chemiluminescence. Research like this may be able to successfully confirm the efficacy of a CAR T-cell therapy, as a future basis for further clinical testing in patients.
References
Fluorescent proteins as tools to aid protein production
A genetically encoded protein tag for control and quantitative imaging of CAR T cell therapy
Sensitivity and Specificity of CD19.CAR-T Cell Detection by Flow Cytometry and PCR
Visualizing CAR-T cell Immunotherapy Using 3 Tesla Fluorine-19 MRI
Pooled screening of CAR T cells identifies diverse immune signaling domains for next-generation immunotherapies
The Chimeric Antigen Receptor Detection Toolkit
Preclinical optimization of a GPC2-targeting CAR T-cell therapy for neuroblastoma
Original created on April 29, 2024, last updated on April 29, 2024
Tagged under: fluorescence, CAR T Cell, fluorescent proteins, validation