Total Internal Refraction Microscopy (TIRFM)
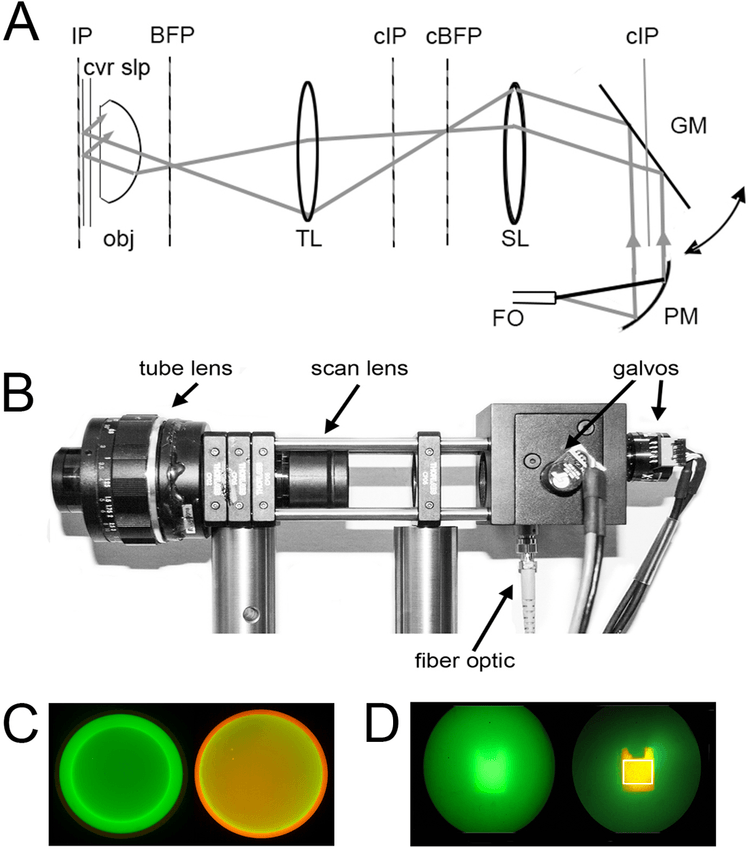
Optical scheme of the spinning spot TIRF illuminator. (A) Optical schematic. (B) Photograph of the spinning spot TIRF illuminator, constructed from Thor Labs optical cage components. (C, D) Photographs taken through the microscope ocular, illustrating the alignment procedure using a rhodamine film/fluorescein solution test specimen. The white box in the right panel outlines the approximate imaging field of the EMCCD camera. Source: Ellefsen KL, Dynes JL, Parker I (2015) Spinning-Spot Shadowless TIRF Microscopy. PLoS ONE.
In a typical setup, fluorophores in an aqueous specimen near the glass interface exhibit an electronic transition at, or close to, the wavelength of the laser beam. These fluorophores become easily excited by the evanescent (electromagnetic) field produced. This evanescent field intensity decays exponentially with distance from the glass substrate, so fluorophores further from the surface do not become excited, thereby greatly reducing the background noise. TIRFM uses a camera, similar to WF microscopy, since specimen excitation occurs across the x-y plane. Imaging speed is determined solely by the frame rate of the camera, and no other factors.
Table of Contents
Advantages
Some advantages of TIRFM are that this technique enables thin regions of a specimen, usually less than 200 nm, to be easily observed. TIRFM allows for the direct monitoring of biomolecules at a single-molecule level in vitro and in living cells, particularly useful for single-molecule nanobioscience. TIRFM enables the possibility of following biochemical reactions, structural changes, movements, and molecular mechanisms of biomolecules in real time.
This method has substantially better optical sectioning than confocal and multiphoton microscopy, and the equipment greatly reduces background noise by limiting the depth of fluorophore excitation to the interface. TIRFM produces high-contrast images of fluorophores near plasma membranes, and reduces cellular photodamage and exposure times.
Limitations
A major limitation of TIRFM lies in that quantitative measurements from fluorescent images are not possible. As sections are confined to a single-plane immediately adjacent to the cover glass, and the evanescent field decays exponentially with distance from the plane, small differences in axial distance of fluorophores from the coverslip will cause large changes in apparent brightness.
Fluorescent signals that are anywhere over 100 nm away from the plane may be too weak to detect. Such a low penetration depth of the evanescent field contributes to limitations in specimens; appropriate specimens should be of specific cell types, like epidermal cells, and must lie flat against the coverslip. Excitation intensity, additionally, is typically uneven across the illuminated area.
Tools: |
Prism-Based TIRFM
In prism-based TIRFM, a prism instead of an objective is used to generate evanescent waves and fluorescence images. Prism-based TIRFM can be used with conventional excitation light sources, like an arc lamp rather than lasers, and can be easily and inexpensively adapted to existing inverted or upright microscopes.
In this method, however, fluorescence must be viewed through the entire specimen, which may degrade the image quality and restrict applications to thin, transparent samples. For successful imaging, specimens must be trapped between the glass coverslip in a thin aqueous layer, making this technique not suitable for live cells.
Objective-Based TIRFM
The objective-based TIRFM approach largely depends on a numerical aperture (NA) objective to produce the evanescent field. Objective-based TIRFM is more common over prism-based methods due to the ease of operation and higher precision.
In this technique, an objective lens directs light to the coverslip-specimen interface. To achieve total internal reflection, the incidence angle must be greater than the critical angle. As the cell cytosol maintains a refractive index of roughly 1.38, the objective NA must exceed this value. Objectives with higher NAs allow for better control of the evanescent field penetration depth, though these objectives are experimentally challenging to create.
Product Ordering Information
Table 1. Cell Navigator® Plasma Membrane Staining kits for live cells.
Product Name ▲ ▼ | Probe ▲ ▼ | Ex/Em (nm) ▲ ▼ | Filter Set ▲ ▼ | Unit Size ▲ ▼ | Cat No. ▲ ▼ |
Cell Navigator® Cell Plasma Membrane Staining Kit *Green Fluorescence* | Cellpaint™ Green | 497/505 | FITC | 500 tests | 22682 |
Cell Navigator® Cell Plasma Membrane Staining Kit *Orange Fluorescence* | Cellpaint™ Orange | 555/573 | TRITC | 500 tests | 22680 |
Cell Navigator® Cell Plasma Membrane Staining Kit *Red Fluorescence* | Cellpaint™ Red | 648/671 | Cy5 | 500 tests | 22681 |