Quest Rhod-4™ Calcium Detection Reagents and Screen Quest™ Rhod-4 NW Calcium Assay Kits
Introduction
Calcium acts as a universal second messenger in a variety of cells. The beginning of life, the act of fertilization, is regulated by Ca2+. Numerous functions of all types of cells are regulated by Ca2+ to a greater or lesser degree. Since the 1920s, scientists have attempted to measure Ca2+, but few were successful due to limited availability of Ca2+ probes. The first reliable measurements of Ca2+ were performed by Ridgway and Ashley by injecting the photoprotein aequorin into the giant muscle fiber of the barnacle. Subsequently, in the 1980s, Tsien and colleagues produced a variety of fluorescent indicators. Among them the rhodamine-based Ca2+ reagents (such as Rhod-2) have been the most valuable longer wavelength dye for measuring Ca2+ with red fluorescence.
Rhod-4™ Calcium Indicators, the Most Sensitive Red Fluorescent Calcium Probe
Although Rhod-2 has been the most popular red fluorescent Ca2+ indicator, its mitochondrial localization and high basal Ca2+ signal in cells have severely limited its cellular applications. In addition, the less optimal excitation of Rhod-2 at 488 nm makes it less robust to use with some instruments (such as FLIPR™) that have only 488 nm excitation light source. Our Rhod-4™ serial calcium detection reagents have been developed to address these limitations of Rhod-2.
The absorption and emission peaks of Rhod-4™ reagents are 530 nm and 555 nm, respectively. Although Rhod-4 has maximum absorption at 530 nm, its absorption at 488 nm is quite strong (see Figure 1). It is quite unique that Rhod-4™ can be well excited with an argon ion laser at 488 nm besides the longer wavelength excitations at 514 nm, 532 nm and 546 nm. Rhod-4™ emits fluorescence (at 555 nm) which increases with the increasing Ca2+. Rhod-4™ is determined to undergo a >200-fold increase in fluorescence upon binding to Ca2+. Because the range of increase in Ca2+ in many cells after stimulation is generally 5- to10-fold, Rhod-4™ is an excellent probe to use with high sensitivity in this region. The Kd of Rhod-4™ is estimated to be 525 nM (22°C, pH 7.0 –7.5), but this value may be significantly influenced by pH, viscosity, and binding proteins in vivo conditions.
Besides their convenient excitation wavelengths and large fluorescence enhancement by calcium, Rhod-4™ is much brighter in cells than Rhod-2 upon agonist stimulation (signal to background ratio) as shown in Figure 2. More importantly, Rhod-4™ is predominantly localized in cytosols unlike Rhod-2 that is mainly localized in mitochondria. Rhod-4™ reagents have a less temperature-dependent cell loading property, giving similar results either at room temperature or 37°C. This characteristic makes Rhod-4™ more robust for HTS applications than Rhod-2 AM.
Table 1. Spectral and Ca2+ Binding Properties of Rhod-4™ Calcium Detection Reagents
Ca2+ Indicator ▲ ▼ | Ex (nm) ▲ ▼ | Em (nm) ▲ ▼ | Kd of Ca2+-Binding ▲ ▼ |
Rhod-4™, AM | 530 | 555 | 525 |
Compared to Rhod-2, our Quest Rhod-4™ calcium detection reagents have the following advantages:
- Convenient Excitation Wavelengths: multiple excitation options @ ~490 nm, 514 nm, 532 nm and 546 nm.
- Much Larger Assay Window: 10 times larger than Rhod-2 AM.
- Flexible Loading: dye loading at room temperature rather than 37°C.
- Versatile Packing Sizes to Meet Your Special Needs: 1 mg; 10 x 50 µg; 20 x 50 µg; HTS packages.
ATP-stimulated calcium responses of endogenous P2Y receptors were measured in CHO-K1 cells with Rhod-4™ AM (Cat# 21120) and Rhod-2 AM (Cat# 21064). CHO-K1 cells were seeded overnight at 50,000 cells/100 µL/well in a Costar 96-well black wall/clear bottom plate. The growth medium was removed, and the cells were incubated with 100 µL of dye loading solution using Rhod-4™ AM (4 µM, A and B) or Rhod-2 AM (4 µM, C and D) for 1 hour in a 37°C, 5% CO2 incubator. The staining solution was replaced with 200 µL HHBS, then the cells were imaged before (A and C) and after (B and D) ATP treatment with a fluorescence microscope (Olympus IX71) using TRITC channel.
Use of Rhod-4™ AM Esters
- Load Cell with Quest Rhod-4™ AM Esters: AM esters are the non-polar esters that readily cross live cell membranes, and rapidly hydrolyzed by cellular esterases inside live cells. AM esters are widely used for loading a variety of polar fluorescent probes into live cell non-invasively. However, cautions must be excised when AM esters are used since they are susceptible to hydrolysis, particularly in solution. They should be reconstituted just before use in high-quality, anhydrous dimethylsulfoxide (DMSO). DMSO stock solutions may be stored desiccated at –20 °C and protected from light. Under these conditions, AM esters should be stable for several months.
The following is our recommended protocol for loading Rhod-4™ AM esters into live cells. This protocol only provides a guideline, and should be modified according to your specific needs.- Prepare a 2 to 5 mM stock solution of Rhod-4™ AM esters in high-quality, anhydrous DMSO.
- On the day of the experiment, either dissolve Rhod-4™ AM in DMSO or thaw an aliquot of the indicator stock solution to room temperature. Prepare a working solution of 1 to 10 µM in Hanks and Hepes buffer (HHBS) or the buffer of your choice with 0.02% Pluronic® F-127. For most of cell lines, Rhod-4™ AM reagents with a concentration ranging from 4-5 uM are recommended. The exact concentration of the indicator required for cell loading must be determined empirically. To avoid any artifacts caused by overloading and potential dye toxicity, it is recommended to use the minimal dye concentration that can generate sufficient signal strength.
Note: The nonionic detergent Pluronic® F-127 is sometimes used to increase the aqueous solubility of Rhod-4™ AM esters. A variety of Pluronic® F-127 solutions can be purchased from AAT Bioquest. - If your cells containing the organic anion-transports, probenecid (1–2.5 mM) or sulfinpyrazone (0.1–0.25 mM) may be added to the cell medium to reduce leakage of the de-esterified indicators.
Note: A variety of ReadiUse™ probenecid including water soluble sodium salt and stabilized solution can be purchased from AAT Bioquest. - Add equal volume of the dye working solution (from Step b or c) into your cell plate.
- Incubate the dye-loading plate at a cell incubator or room temperature for 30 minutes to one hour at room temperature or 37°C.
Note: Decreasing the loading temperature might reduce the compartmentalization of the indictor. - Replace the dye working solution with HHBS or buffer of your choice (containing an anion transporter inhibitor, such as 2.5 mM probenecid, if applicable) to remove excess probes.
- Run the experiments at Ex/Em = 540/590 nm
- Measure Intracellular Calcium Responses: see figure 3.
To determine either the free calcium concentration of a solution or the Kd of a single-wavelength calcium indicator, the following equation is used:
[Ca]free = Kd[F · Fmin]/Fmax · F]
Where F is the fluorescence of the indicator at experimental calcium levels, Fmin is the fluorescence in the absence of calcium and Fmax is the fluorescence of the calcium-saturated probe.
The dissociation constant (Kd) is a measure of the affinity of the probe for calcium. The Ca-binding and spectroscopic properties of fluorescent indicators vary quite significantly in cellular environments compared to calibration solutions. In situ response calibrations of intracellular indicators typically yield Kd values significantly higher than in vitro determinations. In situ calibrations are performed by exposing loaded cells to controlled Ca2+ buffers in the presence of ionophores such as A-23187, 4-bromo A-23187 and ionomycin. Alternatively, cell permeabilization agents such as digitonin or Triton® X-100 can be used to expose the indicator to the controlled Ca2+ levels of the extracellular medium. The Kd value of Quest Rhod-4™ is listed in Table 1 for your reference.
Use of Screen Quest™ Rhod-4 NW Calcium Assay Kits for HTS Applications
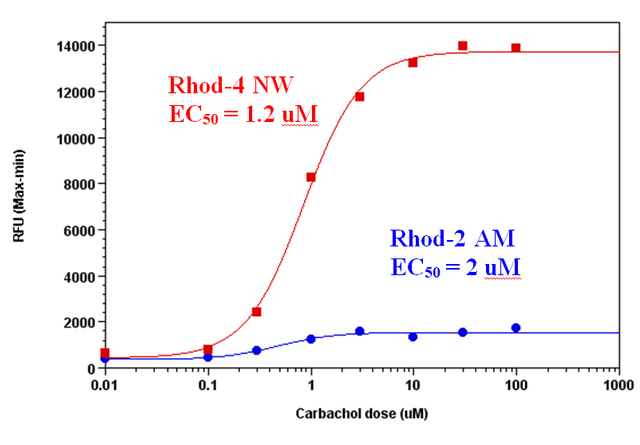
Carbachol Dose Response was measured in HEK-293 cells with Screen Quest™ Rhod-4 NW Assay kit and Rhod-2 AM under the same assay conditions. HEK-293 cells were seeded overnight at 40,000 cells/100 µL/well in a 96-well black wall/clear bottom costar plate. The growth medium was removed, and the cells were incubated with 100 µL of the Screen Quest™ Rhod-4 NW calcium assay kit or Rhod-2 AM for 1 hour at room temperature. Carbachol (25µL/well) was added by NOVOstar™ (BMG LabTech) to achieve the final
Our Screen Quest™ Rhod-4 Calcium Assay Kits have the following advantages for HTS applications:
- Longer Wavelengths: multiple excitations @ 488, 514, 532 &546 nm; maximum emission @ ~555 nm.
- No Wash Required and No Quencher Interference with Your Targets.
- Robust Performance: enable calcium assays that are impossible with Rhod-2 AM.
- Larger Assay Window: 10 times larger than Rhod-2 AM.
Use of Rhod-4™ Salts
Calcium calibration can be carried out by measuring the fluorescence intensity of the salt form (25 to 50 µM in fluorescence microplate readers) of the indicators in solutions with precisely known free Ca2+ concentrations. Calibration solutions can be used based on 30 mM MOPS EGTA Ca2+ buffer. In general, water contains trace amount of calcium ion. It is highly recommended to use 30 mM MOPS + 100 mM KCl, pH 7.2 as buffer system. One can simply make a 0 and 39 µM calcium stock solutions as listed below, and these 2 solutions are used to make a serial solution of different Ca2+ concentrations
- 0 µM calcium: 30 mM MOPS + 100 mM KCl, pH 7.2 buffer + 10 mM EGTA
- 39 µM calcium: 30 mM MOPS + 100 mM KCl, pH 7.2 buffer + 10 mM EGTA + 10 mM CaCl2
To determine either the free calcium concentration of a solution or the Kd of a single-wavelength calcium indicator, the following equation is used:
[Ca]free = Kd[F · Fmin]/Fmax · F]
Where F is the fluorescence intensity of the indicator at a specific experimental calcium level, Fmin is the fluorescence intensity in the absence of calcium and Fmax is the fluorescence intensity of the calcium-saturated probe.
The dissociation constant (Kd) is a measure of the affinity of the probe for calcium. The calcium-binding and spectroscopic properties of fluorescent indicators vary quite significantly in cellular environments compared to calibration solutions. In situ response calibrations of intracellular indicators typically yield Kd values significantly higher than in vitro determinations. In situ calibrations are performed by exposing loaded cells to controlled Ca2+ buffers in the presence of ionophores such as A-23187, 4-bromo A-23187 and ionomycin. Alternatively, cell permeabilization agents such as digitonin or Triton® X-100 can be used to expose the indicator to the controlled Ca2+ levels of the extracellular medium. The Kd values of Fluo-8® reagents are listed in Table 1 for your reference.
Conclusions
Because of the importance of Ca2+ in biology, numerous techniques/methods for analyzing the mechanisms of cellular and/or subcellular Ca2+ activity have been established. Although each method for analyzing Ca2+ activity has certain advantages over the others, each also suffers from drawbacks. With the outstanding properties described above, we believe that Rhod-4™ calcium detection reagents and Screen Quest™ Rhod-4NW Calcium Assay Kits provide new powerful tools for intracellular calcium analysis and monitoring in a variety of biological systems.
As might have been predicted, the interests of many researchers in Ca2+ analysis shifted from the cellular level to the subcellular level. It has been found that Ca2+is not even distributed throughout the whole cell and that intracellular heterogeneity of Ca2+ (such as Ca2+ waves and Ca2+ sparks) is observed in a variety of cells (e.g., oocyte, heart muscle cell, hepatocyte, and exocrine cell). With the advent of the confocal laser scanning microscope (CLSM) in the 1980s and advanced microplate readers dedicated for intracellular calcium detection (such as FLIPR™, FDSS, FlexStation, and NOVOStar™) in 2000s, the measurement of intracellular Ca2+ has accelerated significantly. Confocal laser scanning microscopy and more recently multiphoton microscopy allow the precise spatial and temporal analysis of intracellular Ca2+ signaling at the subcellular level.
Ordering Information
Table 2. Quest Rhod-4™ Product list
Cat No. ▲ ▼ | Product Name ▲ ▼ | Unit Size ▲ ▼ |
21120 | Rhod-4™, AM | 1 mg |
21121 | Rhod-4™, AM | 5 x 50 ug |
21122 | Rhod-4™, AM | 10 x 50 ug |
21123 | Rhod-4™, AM | 20 x 50 ug |
21118 | Rhod-4™, sodium salt | 1 mg |
21119 | Rhod-4™, potassium salt | 1 mg |
21128 | Rhod-4™, sodium salt | 5 x 50 µg |
21129 | Rhod-4™, potassium salt | 5 x 50 µg |
36330 | Screen Quest™ Rhod-4 No Wash Calcium Assay Kit *Medium Removal* | 1 Plate |
36331 | Screen Quest™ Rhod-4 No Wash Calcium Assay Kit *Medium Removal* | 10 Plates |
References
- T. Lock, I. Parker, I.F. Smith, A comparison of fluorescent Ca2+ indicators for imaging local Ca2+ signals in cultured cells, Cell Calcium (2015) October, http://dx.doi.org/10.1016/j.ceca.2015.10.003.
- Rebekah A. Warwick,, Menachem Hanani. Involvement of aberrant calcium signalling in herpetic neuralgia. Experimental Neurology. Volume 277, March 2016, Pages 10–18
- Ji-Hun Seo, Mitsuhi Hirata , Sachiro Kakinoki, Tetsuji Yamaoka and Nobuhiko Yui. Dynamic polyrotaxane-coated surface for effective differentiation of mouse induced pluripotent stem cells into cardiomyocytes. RSC Adv., 2016 March, 6 , 35668-35676. DOI: 1039/C6RA03967G.
- Bansal A,Liu H, Jayakumar MK, Andersson-Engels S, Zhang Y . Quasi-Continuous Wave Near-Infrared Excitation of Upconversion Nanoparticles for Optogenetic Manipulation of C. elegans. 2016 Apr;12(13):1732-43. doi: 10.1002/smll.201503792. Epub 2016 Feb 5.
- Christina M. Ambrosi, Emilia Entcheva. Optogenetic Control of Cardiomyocytes via Viral Delivery. Cardiac Tissue Engineering. 2014 Volume 1181 of the seriesMethods in Molecular Biology pp 215-228.
- Martin Oheim, Marcel van 't Hoff, Anne Feltz, Alsu Zamaleeva, Jean-Maurice Mallet, Mayeul Collot. New red-fluorescent calcium indicators for optogenetics, photoactivation and multi-color imaging. Biochimica et Biophysica Acta 1843 (2014) 2284–2306.
- Erwann Rousseau, Patrick P. Michel, and Etienne C. Hirsch. The Iron-Binding Protein Lactoferrin Protects Vulnerable Dopamine Neurons from Degeneration by Preserving Mitochondrial Calcium Homeostasis . Pharmacol., Dec 2013; 84: 888 - 898.
- Wenjun Xie,Gaetano Santulli, Xiaoxiao Guo, Melanie Gao, Bi-Xing Chen, Andrew R. Marks. Imaging atrial arrhythmic intracellular calcium in intact heart. JMCC, Nov, 2013 volume 64, pages 120-123 DOI: http://dx.doi.org/10.1016/j.yjmcc.2013.09.003.
- Emilia Entcheva. Review: Cardiac optogenetics. Am J Physiol Heart Circ Physiol May 2013; 304: H1179–H1191, 2013.doi:10.1152/ajpheart.00432.2012.
- Alice P. Liou, Yoshitatsu Sei, Xilin Zhao, Jianying Feng, Xinping Lu, Craig Thomas, Susanne Pechhold, Helen E. Raybould, and Stephen A. Wank . The extracellular calcium-sensing receptor is required for cholecystokinin secretion in response toL-phenylalanine in acutely isolated intestinal I cells. Am J Physiol Gastrointest Liver Physiol. 2011; 300: G538 - G546.
- Jia Z, Valiunas V, Lu Z, Bien H, Liu H, Wang HZ, Rosati B, Brink PR, Cohen IS, Entcheva E., Stimulating cardiac muscle by light: cardiac optogenetics by cell delivery. Circ Arrhythm Electrophysiol. 2011 Oct;4(5):753-60. Epub 2011 Aug 9.
- Wenjun Xie,Gaetano Santulli, Xiaoxiao Guo, Melanie Gao, Bi-Xing Chen, Andrew R. Marks. Imaging atrial arrhythmic intracellular calcium in intact heart. JMCC, Nov, 2013 volume 64, pages 120-123 DOI: http://dx.doi.org/10.1016/j.yjmcc.2013.09.003.
- Iguchi M,Kato M, Nakai J, Takeda T, Matsumoto-Ida M, Kita T, Kimura T, Akao M. Direct monitoring of mitochondrial calcium levels in cultured cardiac myocytes using a novel fluorescent indicator protein, GCaMP2-mt. Int J Cardiol. 2012 Jul 12;158(2):225-34. doi: 10.1016/j.ijcard.2011.01.034. Epub 2011 Feb 4.
- Nishitani, Wagner S. Molecular mechanisms involved in cell response to mechanical forces. 2011.
Original created on January 10, 2022, last updated on November 8, 2022
Tagged under: