An introduction to lysyl oxidase and its quantification
The improper regulation of lysyl oxidase (LOX) has been associated with several fibrotic diseases in humans. Elevated, or overregulated, levels of LOX activity have been linked with the pathogenesis of conditions such as pulmonary fibrosis, systemic sclerosis and liver cirrhosis.1, 5On the other hand, downregulation of LOX activity has been found in the development of aortic diseases such as aortic aneurysms and bone diseases such as osteolathyrism.12, 16, 18More recently, LOX has been the focus of study due to its potential role in the metastasis of various cancers. For example, recent studies in mouse models have shown that LOX is a mediator for the metastasis of human breast cancer cells.5
Because of its importance in biological processes, and its role in the pathogenesis of various human conditions, it is critical for research that there be specific and sensitive methods to quantify LOX activity. Here, two methods are reviewed: a radiometric method using tritium and a fluorimetric method using Amplite® Red.
Because of its importance in biological processes, and its role in the pathogenesis of various human conditions, it is critical for research that there be specific and sensitive methods to quantify LOX activity. Here, two methods are reviewed: a radiometric method using tritium and a fluorimetric method using Amplite® Red.
Overview
Lysyl oxidase belongs to a family of enzymes known as amine oxidases. This family of enzymes catalyzes the oxidation of amines to aldehydes. A generic form of this reaction is shown below:
RCH2NH2 + H2O + O2 ‡ RCHO + NH3 + H2O2
(equation 1)
An important thing to note is the byproduct of amine oxidation, which is a molecule of hydrogen peroxide. This is important because the production of hydrogen peroxide can be coupled to an enzymatic reaction, such as H2O2-dependent HRP, to monitor LOX activity.
In organisms, amine oxidases are used to regulate the levels of many biologically important amines. For example, histamine, the well-known molecule involved in local immune responses, is broken down by the amine oxidase, diamine oxidase (DAO). In the case of LOX, the oxidation target is the amine group on lysine side chains.
Synthesis and activity
In cells, lysyl oxidase is typically first synthesized as a 50 kDa protein precursor called prolysyl oxidase. This inactive form of the protein is then glycosylated in the Golgi Complex, forming an N-glycosylated proenzyme. After glycosylation, it is transported via vesicles to the cell membrane, wherein it is secreted into the extracellular matrix (ECM). While not fully established, evidence suggests that it is during this secretion into the ECM that LOX becomes activated. In particular, studies have pointed to cell surface peptidases as the enzymes which cleave the prolysyl oxidase peptide into the catalytically active, 32 kDa lysyl oxidase form.22, 23
In terms of activity, lysyl oxidase requires two cofactors to function: copper and lysyl tyrosyl quinone (LTQ). As with many copper amine oxidases (CAO), the bound copper, Cu(II), serves to spontaneously generate the quinone cofactor, creating a redox active site in the enzyme. For LOX, the quinone cofactor is LTQ, which is formed through the crosslinking of a tyrosine residue and a lysyl side chain.8, 24
Functionally, lysyl oxidase is involved in the crosslinking of collagen or elastin in the extracellular matrix. The formation of crosslinks between collagen fibers, or elastin fibers, by LOX typically occurs in a two-step process. First, LOX catalyzes the oxidative deamination of lysyl residues on tropopcollagen and tropoelastin. This reaction produces peptidyl α-aminoadipic-δ-semialdehyde, which is also called allysine. In the second step, the produced aldehyde intermediary, allysine, undergoes a spontaneous condensation reaction. This reaction results in the crosslinking of collagen, or elastin, lysyl sidechains.21 In collagen, crosslinking occurs between three lysine residues, forming pyridinoline (also known as hydroxylysyl pyridinoline). In elastin, crosslinking occurs between four lysine residues, forming desmosine.
Cancer metastasis
As discussed previously, lysyl oxidase is functionally important in collagen, or elastin, crosslinking. As such, it can also be inferred that LOX plays an important role in the structural integrity and remodeling of the extracellular matrix, of which collagen and elastin are critical compounds. This is supported by studies of various human fibrotic diseases, in which LOX is improperly regulated. But perhaps what is not as apparent is the role of LOX in the metastasis of tumors and cancer cells. And yet, several well-conducted studies on human breast cancer cell lines demonstrate why such may be the case. That is, why LOX activity may be central to the metastasis of cancer cells.
A study conducted by Dawn et al. compared the expression of LOX (and lysyl oxidase-like enzymes LOXL, LOXL2, LOXL3 and LOXL4) in two different human breast cancer cell lines. One was MDA-MB-231, a highly invasive/metastatic cell line, while the other was MCF-7, a poorly invasive/nonmetastatic cell line. What the research group found was that LOX and LOXL2 activity was strongly associated with the invasive MDA-MB-231 cell line while none of the LOX or LOXL proteins were highly expressed in the poorly invasive MCF-7 cell line. Blocking LOX expression with an antisense oligonucleotide or inhibiting LOX activity with an irreversible inhibitor, β-aminopropionitrile, also decreased invasive activity in the MDA-MB-231 cell line.14 These results were replicated in and supported by a plethora of studies since.3, 6, 7, 10, 20
A paper by Cox et al. proposes a theory for why LOX activity may be so closely tied to tumor metastasis. The theory is that LOX activity, whether normal expression or overexpression, creates a fibrotic milieu that enhances tumor colonization and outgrowth of tumor cells. That is, LOX activity creates a scaffold upon which tumor cells can spread.4 This idea garners further support from studies which show that inhibition of LOX does not inhibit primary tumor growth, but does restrict secondary (i.e. metastatic) tumor activity. In a biological context, this theory is very logical as it is, in principle, the same concept as soft tissue wound healing, wherein myofibroblasts deposit fibers such as collagen to promote the division and spread of epithelial and endothelial cells over an injury site.25
Tritium release end point assay
One of the first, and perhaps still the most sensitive, lysyl oxidase activity assays is the tritium release end point assay. Here, end point assay simply refers to a single quantification after a specified incubation period, as opposed to a kinetic assay, which quantifies activity at several instances over a duration of time. With the tritium release end point assay, the sample of interest (i.e. a tissue or organ culture) is first incubated in a lysine-deficient medium, followed by a medium with tritium-labeled lysine in the presence of β-aminopropionitrile (BAPN). This promotes the exhaustion of endogenous, non-labeled lysine and allows for pulse labeling with tritium-labeled lysine. After a specified incubation period, the sample of interest is lysed and the tritium containing solution is collected by vacuum distillation. Quantification of the tritiated water ([3H]OH) yields measurements proportional to LOX activity. This assay works on the principle that LOX-catalyzed oxidative deamination of lysine residues releases tritium into solution at a one to one rate (see equation 1). 13, 15, 17
The tritium release end point assay is the most sensitive assay for LOX activity because it is a direct measurement of the oxidative deamination product, that is, tritiated water. However, it has three big disadvantages. First, it is very inconvenient and labor intensive to conduct, requiring vacuum distillation of each sample. This makes it an impractical method for medium to large scale testing. Second, it requires the use of radioactive isotopes. While not dangerous through external exposure, ingestion, inhalation or absorption through the skin can pose a radioactive hazard. Third, and finally, special equipment is required to conduct radioactive counting of the tritiated water, which may not be available to many researchers. Because of these disadvantages, a spectroscopic method for monitoring LOX activity should be strongly considered.
Amplite® fluorimetric lysyl oxidase activity assay
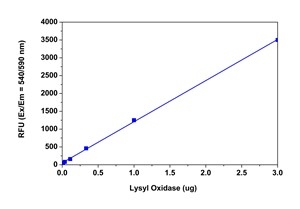
Lysyl oxidase dose response was measured on a solid black 96-well with Amplite® Fluorimetric LysylOxidase Assay Kit (Cat# 15255) using a Gemini fluorescence microplate reader (Molecular Devices). As low as 40 ng/mL of lysyl oxidase can be detected with 30 minutes incubation (n=3).
One important thing to note is that, in principle, spectroscopic assays, such as AmLOX™, will be less sensitive than tritium release end point assays. This is because spectroscopic assays are indirect measurement methods which utilize a secondary detection system (i.e. H2O2-dependent HRP) whereas the tritium release end point assay is a direct measurement method that quantifies the byproduct of the LOX reaction. Thus, in theory, AmLOX™ will not achieve the same sensitivity as tritium-based assay. In practice, however, AmLOX™ shows comparable results to tritium release end point assays. Our testing has shown that AmLOX™ is able to detect sub-ng/well of lysyl oxidase, a detection limit which more than satisfies the requirement for most applications.
AmLOX™ demonstrates comparable experimental sensitivity to tritium release end point assays. But AmLOX™ has several other distinct features that make it a better overall assay choice than tritium-based ones. First, AmLOX™ does not use any radioactive or highly toxic chemicals. Second, AmLOX™ can be paired with very standard microplate instrumentation found in many labs. Elaborating on this point, AmLOX™ can actually be read in two different modes. It can be used as a colorimetric assay in conjunction with an absorbance microplate (read at 576 nm). It can also be used as a fluorimetric assay and paired with a fluorescence microplate reader (using 540 nm as the excitation and 590 nm as the emission). This offers researchers extra flexibility in terms of experimental design. Finally, unlike tritium-based assays, there is no laborious vacuum distillation required. This assay can be completely and conveniently executed in a standard 96- or 384- well microplate.
A sample protocol has been provided below:
- Prepare sample in microplate beforehand
- Add 50 µL of AmLOX™ working solution into each well
- Incubate at 37 °C for 10 to 30 minutes, protected from light
- Read fluorescence using a fluorescence microplate reader (using Ex = 540 nm and Em = 590 nm)
Table 1. LOX Assays and Related Products
Cat No. ▲ ▼ | Product Name ▲ ▼ | Ex (nm) ▲ ▼ | Em (nm) ▲ ▼ |
15255 | Amplite® Fluorimetric Lysyl Oxidase Assay Kit *Red Fluorescence* | 571 | 584 |
13512 | Amplite® MMP-3 Activity Assay Kit *Green Fluorescence* | 494 | 515 |
13510 | Amplite® Universal Fluorimetric MMP Activity Assay Kit *Green Fluorescence* | 494 | 515 |
13511 | Amplite® Universal Fluorimetric MMP Activity Assay Kit *Red Fluorescence* | 545 | 572 |
References
- Aumiller, V., Strobel, B., Romeike, M., Schuler, M., Stierstorfer, B. E., & Kreuz, S. (2017). Comparative analysis of lysyl oxidase (like) family members in pulmonary fibrosis.Scientific reports,7(1), 149.
- Barry-Hamilton, V., Spangler, R., Marshall, D., McCauley, S., Rodriguez, H. M., Oyasu, M., ... & Garcia, C. A. (2010). Allosteric inhibition of lysyl oxidase"like-2 impedes the development of a pathologic microenvironment. Nature medicine, 16(9), 1009.
- Bondareva, A., Downey, C. M., Ayres, F., Liu, W., Boyd, S. K., Hallgrimsson, B., & Jirik, F. R. (2009). The lysyl oxidase inhibitor, β-aminopropionitrile, diminishes the metastatic colonization potential of circulating breast cancer cells. PloS one, 4(5), e5620.
- Cox, T. R., Bird, D., Baker, A. M., Barker, H. E., Ho, M. W., Lang, G., & Erler, J. T. (2013). LOX-mediated collagen crosslinking is responsible for fibrosis-enhanced metastasis. Cancer research, 73(6), 1721-1732.
- Cox, T. R., & Erler, J. T. (2011). Remodeling and homeostasis of the extracellular matrix: implications for fibrotic diseases and cancer.Disease models & mechanisms,4(2), 165-178.
- Cox, T. R., Gartland, A., & Erler, J. T. (2016). Lysyl oxidase, a targetable secreted molecule involved in cancer metastasis. Cancer research, 76(2), 188-192.
- Cox, T. R., Rumney, R. M., Schoof, E. M., Perryman, L., Hye, A. M., Agrawal, A., ... & Huggins, I. D. (2015). The hypoxic cancer secretome induces pre-metastatic bone lesions through lysyl oxidase. Nature, 522(7554), 106.
- DuBois, J. L., & Klinman, J. P. (2005). Mechanism of post-translational quinone formation in copper amine oxidases and its relationship to the catalytic turnover. Archives of biochemistry and biophysics, 433(1), 255-265.
- Erler, J. T., Bennewith, K. L., Cox, T. R., Lang, G., Bird, D., Koong, A., ... & Giaccia, A. J. (2009). Hypoxia-induced lysyl oxidase is a critical mediator of bone marrow cell recruitment to form the premetastatic niche. Cancer cell, 15(1), 35-44.
- Erler, J. T., Bennewith, K. L., Nicolau, M., Dornhöfer, N., Kong, C., Le, Q. T., ... & Giaccia, A. J. (2006). Lysyl oxidase is essential for hypoxia-induced metastasis. Nature, 440(7088), 1222.
- Gupta, G. P., & Massagué, J. (2006). Cancer metastasis: building a framework. Cell, 127(4), 679-695.
- Hammond, M. A., & Wallace, J. M. (2015). Exercise prevents [beta]-aminopropionitrile-induced morphological changes to type I collagen in murine bone. BoneKEy reports, 4.
- Kagan, H. M., & Sullivan, K. A. (1982). [35] Lysyl oxidase: Preparation and role in elastin biosynthesis. In Methods in enzymology (Vol. 82, pp. 637-650). Academic Press.
- Kirschmann, D. A., Seftor, E. A., Fong, S. F., Nieva, D. R., Sullivan, C. M., Edwards, E. M., ... & Hendrix, M. J. (2002). A molecular role for lysyl oxidase in breast cancer invasion. Cancer research, 62(15), 4478-4483.
- Konishi, A., Iguchi, H., Ochi, J., Kinoshita, R., Miura, K., & Uchino, H. (1985). Increased lysyl oxidase activity in culture medium of nonparenchymal cells from fibrotic livers. Gastroenterology, 89(4), 709-715.
- Lee, V. S., Halabi, C. M., Hoffman, E. P., Carmichael, N., Leshchiner, I., Lian, C. G., ... & Stitziel, N. O. (2016). Loss of function mutation in LOX causes thoracic aortic aneurysm and dissection in humans.Proceedings of the National Academy of Sciences,113(31), 8759-8764.
- Li, W., Nellaiappan, K., Strassmaier, T., Graham, L., Thomas, K. M., & Kagan, H. M. (1997). Localization and activity of lysyl oxidase within nuclei of fibrogenic cells. Proceedings of the National Academy of Sciences, 94(24), 12817-12822.
- Mäki, J. M., Räsänen, J., Tikkanen, H., Sormunen, R., Mäkikallio, K., Kivirikko, K. I., & Soininen, R. (2002). Inactivation of the lysyl oxidase gene Lox leads to aortic aneurysms, cardiovascular dysfunction, and perinatal death in mice. Circulation, 106(19), 2503-2509.
- Mecham, R. (2018) Methods in Extracellular Matrix Biology. Retrieved from https://books.google.com
- Payne, S. L., Fogelgren, B., Hess, A. R., Seftor, E. A., Wiley, E. L., Fong, S. F., ... & Kirschmann, D. A. (2005). Lysyl oxidase regulates breast cancer cell migration and adhesion through a hydrogen peroxide"mediated mechanism. Cancer research, 65(24), 11429-11436.
- Pinnell, S. R., & Martin, G. R. (1968). The cross-linking of collagen and elastin: enzymatic conversion of lysine in peptide linkage to alpha-aminoadipic-delta-semialdehyde (allysine) by an extract from bone. Proceedings of the National Academy of Sciences, 61(2), 708-716.
- Rucker, R. B., Kosonen, T., Clegg, M. S., Mitchell, A. E., Rucker, B. R., Uriu-Hare, J. Y., & Keen, C. L. (1998). Copper, lysyl oxidase, and extracellular matrix protein cross-linking. The American journal of clinical nutrition, 67(5), 996S-1002S.
- Smith-Mungo, L. I., & Kagan, H. M. (1998). Lysyl oxidase: properties, regulation and multiple functions in biology. Matrix biology, 16(7), 387-398.
- Wang, S. X., Nakamura, N., Mure, M., Klinman, J. P., & Sanders-Loehr, J. (1997). Characterization of the native lysine tyrosylquinone cofactor in lysyl oxidase by Raman spectroscopy. Journal of Biological Chemistry, 272(46), 28841-28844.
- Wynn, T. A. (2008). Cellular and molecular mechanisms of fibrosis. The Journal of pathology, 214(2), 199-210.