Selective Probes for Studying Mitochondrial Functionality
AAT Bioquest offers a broad selection of tools designed to investigate mitochondrial morphology and functionality, and ROS imaging. The following discussion highlights assay reagents and kits optimized for mitochondrial ROS imaging, as well as, exploring a convenient method for improving Rhod-2 sensitivity at detecting mitochondrial calcium. For a detailed overview of the various tools we provide to monitor mitochondrial morphology and functionality refer to the Table 1-2.
Mitochondria ROS Imaging
A byproduct of mitochondrial oxidative phosphorylation is ROS. These chemically reactive, oxygen-containing species are released into the cytoplasm to promote redox signaling. However, an improper balance of ROS production-detoxification leads to oxidative stress (ROS overproduction), which is characterized by the potentially damaging levels of intracellular ROS. Because ROS overproduction is detrimental to mitochondria it must be neutralized in order maintain normal cellular homeostasis. Failure to do so will result in damage to the organelle's lipids, proteins and DNA, and can induce cell damaging activities such as the induction of the mitochondrial permeability transition pore (mPTP). Opening of the mPTP channel is accompanied by an increase in the inner membrane permeability, the dissipation of the mitochondrial membrane potential, and a decrease in ATP production. The changes induced by the opening of mPTP can induce cell death.
Consequently, all of these different aspects of mitochondrial ROS have also been implicated in various pathologies including ischemia reperfusion injury, myocardial infarction, and chronic disorders (e.g., neurodegenerative disease, or type-2 diabetes). As such, the detection and quantification of mitochondrial ROS is important to understanding proper cellular redox regulation and the impact of its dysregulation on various cellular pathways and pathologies.
Superoxide Radical
Mitochondria are potent producers of cellular superoxide (•-O2−), a by-product of mitochondrial respiration (most notably by Complex I and Complex III). Superoxide is the predominant ROS in mitochondria. Superoxide, at low to moderate levels, is critical for the proper regulation of many essential cellular processes including gene expression and signal transduction. However, studies have shown mice deficient for mitochondrial superoxide dismutase (enzyme that neutralizes superoxide radicals) die due to neurodegeneration, cardiomyopathy and lactic acidosis (pathologies commonly correlated with elevated superoxide levels) (Muller et al. 2007). Therefore, investigating mitochondrial superoxide (exogenous or endogenous) levels are of great interest to study these medically relevant pathologies.
Cell Meter™ Fluorimetric Mitochondrial Superoxide Activity Assay Kit
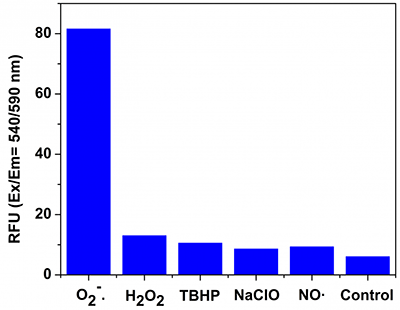
MitoROS™ 520 is unique in that it generates a strong green fluorescence when it is oxidized by superoxide and binds to DNA (Figure 2). Utilization of the green spectrum creates enough spectral separation from other red ROS indicators, such as MitoROS™ OH 580 (a hydroxyl radical indicator) making it suitable for the simultaneous monitoring of superoxide and hydroxyl radical levels. Or one can investigate mitochondrial calcium uptake and ROS production using MitoROS™ 520 and Rhod-2, a red calcium indicator.
Hydroxyl Radical
The hydroxyl radical (•-OH) is another byproduct of oxidative metabolism and is the most reactive ROS. Hydroxyl radicals can produce DNA damage and cause significant subcellular damage to organelles. Therefore, sensitive and selective detection of intracellular •-OH is vital to understanding cellular redox and the impact of hydroxyl radicals on dysregulation in cells. While a variety of •-OH fluorogenic sensors are commercially available, many suffer from rapid photobleaching, short emission wavelengths, and non-selective reactions with other ROS species. All these factors have limited the applications in cells and tissues. To resolve these limitations, AAT Bioquest has dedicated extensive research and development in producing MitoROS™ OH580, a robust and sensitive •-OH fluorogenic sensor.
Cell Meter™ Mitochondrial Hydroxyl Radical Detection Kit
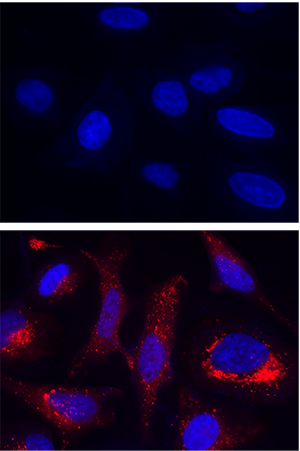
Mitochondrial Calcium Imaging
Calcium uptake and efflux in mitochondria is important in regulating cytosolic calcium concentrations and metabolic processes. Mitochondrion possess the ability to rapidly take up calcium, primarily through the mitochondrial calcium uniporter (MCU) located on the inner mitochondrial membrane. Calcium influx into the mitochondrial matrix is dependent upon the organelles electrochemical gradient for calcium. This gradient is established and maintained by mitochondrial respiration, while the mitochondrial sodium-calcium antiporter is responsible for sustaining a low resting intramitochondrial calcium concentration.
Intramitochondrial calcium plays an intimate role in regulating mitochondrial metabolism by targeting three key rate limiting dehydrogenases of the citric acid cycle (CAC). Calcium directly binds to the enzyme complexes and activates α-ketoglutarate and isocitrate dehydrogenases. Pyruvate dehydrogenase activation is mediated through a calcium-dependent dephosphorylation step. Calcium activation of these dehydrogenase enzymes in mitochondria leads to an increase in NADH and the production of ATP. Disruption of mitochondrial calcium uptake alters the spatiotemporal properties of cytosolic calcium signaling and suppresses mitochondrial metabolism, which leads to a decrease in ATP production. This type of disruption of mitochondrial calcium uptake has been found to cause diseases such as chronic fatigue.
Rhod-2: Mitochondrial Calcium Indicator
Rhod-2, AM can be used to measure changes in mitochondrial calcium inside cells. Rhod-2, AM is a rhodamine-based calcium indicator that is conjugated with AM esters to become cationic.
This is advantageous for two reasons: 1) the cationic charge on Rhod-2, AM promotes the potential driven accumulation of Rhod-2 within mitochondria via mitochondria membrane potential 2) AM esters facilitate the diffusion of Rhod-2 across the cytoplasmic membrane as well as the mitochondrial membrane. Once inside the mitochondria, non-specific intracellular esterases remove the AM groups, which traps Rhod-2 inside the mitochondria. Rhod-2 exhibits a significant increase in fluorescence intensity upon association with calcium ions.
Rhod-2 has a fluorescence excitation and emission maxima at 549 nm and 578 nm, respectively. This characteristically long-wavelength makes it suitable for cellular and tissue imaging which have relatively high autofluorescence. This is an advantage for detecting calcium release generated by photoreceptors and photoactivatable chelators.
One of the disadvantages of Rhod-2 AM is its susceptibility to background interference. Non-specific intracellular esterases are not unique to mitochondria and are present throughout the cytosol. When Rhod-2 AM is loaded into cells, the indicators can be activated and retained within the cytosol before it can be sequestered within mitochondria. A solution for improving Rhod-2 sensitivity is to reduce it to dihydrorhod-2 AM prior to use.
Improving Rhod-2 Sensitivity: Method to Reduce Rhod-2 to Dihydrorhod-2
Reduction of Rhod-2 AM to dihydrorhod-2 AM enhances its mitochondrial localization and reduces background interference. This change improves sensitivity because it requires a two-part activation process. The non-fluorescent dihydrorhod-2 AM can only exhibit calcium-dependent fluorescence after oxidation and AM cleavage in the mitochondria. Residual dihydrorhod-2 retained in the cytosol, due to AM cleavage, cannot be taken up by the mitochondria for oxidation and will remain non-fluorescent even in the presence of cytosolic calcium. Reduction of Rhod-2 AM to dihydrorhod-2 AM can be readily achieved using the protocol below:
- Dissolve 50 µg of Rhod-2 AM in 100 µL of anhydrous dimethylsulfoxide (DMSO).
- Add a small excess (the smallest amount solid that can be practically transferred is sufficient enough) of solid sodium borohydride (NaBH4 ).
- Incubate for 10 minutes or until the reaction mixture appears colorless.
- Use the reaction solution in DMSO (about 0.4 mM dihydrorhod-2 AM) directly for cell loading according to usual AM ester loading protocols.
*Note: Dihydrorhod-2 AM will spontaneously and rapidly revert to its oxidized form, this is indicated by reappearance of color in your stock solution. So, experiments using dihydrorhod-2 AM should be carried out immediately after preparation.
Appendix: AAT Bioquest Mitochondrial Tools
The following tables provide a summary of the AAT Bioquest's mitochondrial tools and stains as well as their targets.
Table 1. AAT Bioquest tools to study mitochondrial morphology.
Probe | Catalog# | Ex/Em (nm) | Fixable | Application | Principle |
MitoLite™ Blue FX490 | 22674 | 350/490 | Yes | Live cell | Cationic fluorogenic probe sequestered by functioning mitochondria via the mitochondrial membrane potential gradient (ΔΨM). MitoLite™ FX dyes retain fluorescent staining pattern after fixation and permeabilization. |
MitoLite™ Green EX 488 | 22675 | 498/520 | No | ||
MitoLite™ Green FM | 22695 | 491/513 | Yes | ||
MitoLite™ Orange EX405 | 22679 | 399/550 | No | ||
MitoLite™ Orange FX570 | 22676 | 545/575 | Yes | ||
MitoLite™ Red FX600 | 22677 | 575/600 | Yes | ||
MitoLite™ Deep Red FX660 | 22678 | 640/659 | Yes | ||
MitoLite™ NIR Red FX690 | 22690 | 660/692 | Yes |
Table 2. AAT Bioquest tools to study mitochondrial functionality
|
References
- Bowser, D N et al. Role of Mitochondria in Calcium Regulation of Spontaneously Contracting Cardiac Muscle Cells. Biophysical Journal75.4 (1998): 2004"2014. Print.
- Carafoli, E. The Role of Mitochondria in the Contraction-Relaxation Cycle and Other Ca2+-Dependent Activities of Heart Cells. Recent Adv. Stud. Cardiac. Struct. Metab. 5 (1975) 151-163.
- Collins, Y., et al. Mitochondrial Redox Signalling at a Glance. Journal of Cell Science, vol. 125, no. 7, Jan. 2012, pp. 1837"1837., doi:10.1242/jcs.110486.
- Duchen, Michael R. Mitochondria and Calcium: From Cell Signalling to Cell Death. The Journal of Physiology529.Pt 1 (2000): 57"68.PMC. Web. 20 Mar. 2018.
- Griffiths, Elinor J., and Guy A. Rutter. Mitochondrial Calcium as a Key Regulator of Mitochondrial ATP Production in Mammalian Cells. Biochimica Et Biophysica Acta (BBA) - Bioenergetics, vol. 1787, no. 11, 2009, pp. 1324"1333., doi:10.1016/j.bbabio.2009.01.019.
- Kirichok, Yuriy, et al. The Mitochondrial Calcium Uniporter Is a Highly Selective Ion Channel. Nature, vol. 427, no. 6972, 2004, pp. 360"364., doi:10.1038/nature02246.
- Lambert, David G., and Richard D. Rainbow.Calcium Signaling Protocols. Humana Press, 2013.
- Muller, Florian L., et al. Trends in Oxidative Aging Theories. Free Radical Biology and Medicine, vol. 43, no. 4, 2007, pp. 477"503., doi:10.1016/j.freeradbiomed.2007.03.034.
- Perry, Seth W. et al. Mitochondrial Membrane Potential Probes and the Proton Gradient: A Practical Usage Guide. BioTechniques50.2 (2011): 98"115.PMC. Web. 20 Mar. 2018.
- Shadel, GeraldS., and TamasL. Horvath. Mitochondrial ROS Signaling in Organismal Homeostasis. Cell, vol. 163, no. 3, 2015, pp. 560"569., doi:10.1016/j.cell.2015.10.001.
- Zhang, X., and F. Gao. Imaging Mitochondrial Reactive Oxygen Species with Fluorescent Probes: Current Applications and Challenges. Free Radical Research, vol. 49, no. 4, 2015, pp. 374"382., doi:10.3109/10715762.2015.1014813.