Lithium Ion Detection & Analysis
Lithium (Li) is found in small amounts in nearly all igneous rocks and in the waters and minerals of many natural springs. Most lithium is cultivated from brines that yield lithium carbonate when treated with sodium carbonate. Lithium's metallic form is produced by the electrolysis of molten lithium chloride and potassium chloride, and is commonly found in everyday life. Perhaps most importantly, rechargeable lithium and lithium ion (Li+) batteries have been widely used for cell phones, laptops, cameras and electric vehicles. These batteries are also used in some non-rechargeable batteries for some biomedical applications, like heart pacemakers, hearing aids, surgical tools, defibrillators, infusion pumps, and monitors. Likewise, lithium alloys are made with aluminum and magnesium, and are used as armor plating for items like aircrafts, bikes and high-speed trains. Various lithium compounds can be found in a number of other places, including glass ceramics (lithium oxide), air conditioning and industrial drying systems (lithium chloride and lithium bromide), high-temperature lubricant (lithium stearate), and for hydrogen fuel storage (lithium hydride).
Though lithium has no known natural biological role and its mechanism on the brain is not fully understood, lithium salts (namely, lithium carbonate), are known to impact neurological function. Lithium salts are used for the treatment of manic-depressive psychosis and bipolar disorders, and have been shown as potent therapeutic agents for deficits caused by traumatic brain injury. Lithium-based medications have also been used to treat skin disease, amyotrophic lateral sclerosis and Alzheimer disease, and recently the antiviral activity of lithium-based drugs have been observed against DNA-type viruses. In small doses, lithium ion drug delivery does not present any clinical effect, however lithium can be harmful to the nervous system, liver and brain, or even lethal where concentrations in the blood exceed 20 mg/L. Due to side effects and toxicity, rapid and reliable determination of lithium ion concentration in biofluids is needed to ensure the safety of the therapeutic used. For patients that receive lithium-based pharmaceuticals, the concentration of lithium in the patient's blood or saliva must be frequently monitored due to potential toxicity. This presents a need for a simple, cost effective, point-of-care lithium detection.
As stated before, the significance of lithium quantitation methods reaches just beyond biological domains, and extends into environmental applications. The expanding use of lithium products, in particular lithium ion batteries, is likely to bring more environmental exposure through eco-contamination by leaching into landfills. Metal contamination in water is a critical and growing issue in light of the potential risks to human wellbeing and the wider environment in general.
Though lithium has no known natural biological role and its mechanism on the brain is not fully understood, lithium salts (namely, lithium carbonate), are known to impact neurological function. Lithium salts are used for the treatment of manic-depressive psychosis and bipolar disorders, and have been shown as potent therapeutic agents for deficits caused by traumatic brain injury. Lithium-based medications have also been used to treat skin disease, amyotrophic lateral sclerosis and Alzheimer disease, and recently the antiviral activity of lithium-based drugs have been observed against DNA-type viruses. In small doses, lithium ion drug delivery does not present any clinical effect, however lithium can be harmful to the nervous system, liver and brain, or even lethal where concentrations in the blood exceed 20 mg/L. Due to side effects and toxicity, rapid and reliable determination of lithium ion concentration in biofluids is needed to ensure the safety of the therapeutic used. For patients that receive lithium-based pharmaceuticals, the concentration of lithium in the patient's blood or saliva must be frequently monitored due to potential toxicity. This presents a need for a simple, cost effective, point-of-care lithium detection.
As stated before, the significance of lithium quantitation methods reaches just beyond biological domains, and extends into environmental applications. The expanding use of lithium products, in particular lithium ion batteries, is likely to bring more environmental exposure through eco-contamination by leaching into landfills. Metal contamination in water is a critical and growing issue in light of the potential risks to human wellbeing and the wider environment in general.
Traditional Detection Approaches
Analogous to detection of other alkali metals, the development of a selective lithium ion quantitation assay remains challenging, particularly for medical and biological applications. Traditionally, flame emission photometry (FEP) was used to detect lithium ions, as it provided a characteristic red flame color with an emission at 670 nm.
In one historically significant experiment by Riley and Tongudai (1964) 30 samples of seawater from different oceans were analyzed to determine lithium ion contents through FEP. As the complex composition of seawater prevented the direct determination of lithium ion concentration, a pre-treatment using ion exchange chromatography was included. In conclusion, it was found that lithium ion concentration was proportional to the concentration of chloride across samples. Alternative methods have also been used to determine serum concentrations of lithium salts, including atomic emission, and absorbance spectrophotometry which measure the light intensity of excited atoms, typically in the visible to UV region of the electromagnetic spectrum.
Other traditional approaches to lithium quantitation have utilized ion-selective electrodes, which measure the electric potential difference through selective ion binding, as well as X-ray fluorescence spectrometry which can determine the elemental composition of a material. All of these approaches, however, are time consuming, require excessive sample preparation, and do not offer high throughput capabilities. The instrumentation for these techniques is also not available in most hospital laboratories, which makes frequent, near-patient monitoring impossible.
Colorimetric Detection
One widely accepted method of lithium ion quantitation is through the use of commercially available colorimetric kits. These kits are simple, one-step, and can use undiluted plasma, serum, or other biological fluids as starting material. Colorimetric lithium detection kit materials are commonly ready to use as supplied, including the lithium assay buffer, standard, probe solution, and sodium masking solution. In experimentation, the standard curve should be prepared according to the manufacturer's instructions alongside the assay to ensure accurate quantitation of lithium.
In a typical protocol, first, the aqueous biological starting material is added to each well. Next, a sodium masking solution is added which prevents sodium ions present in the sample from interfering with the reaction. Then, a buffer and probe solution are added and the tray is incubated. The probe is lithium-selective and bi-chromatic, which means it undergoes an absorbance change at two distinct wavelengths upon binding. Finally, the absorbance should be measured at both 540 nm and 630 nm, and the ratio of the two measurements can be used to calculate lithium ion concentration within the sample. Most kits can detect lithium concentration as low as 100 µM in human serum, and endogenous ions do not interfere with the assay. The colorimetric assay requires minimal time to perform, is adaptable to high-throughput applications, and has a linear range from 0.5-10 nmol of lithium ions, corresponding to 0.1-2 mM in serum.
Tools: | |
Newer Techniques
Fluorescent Indicators
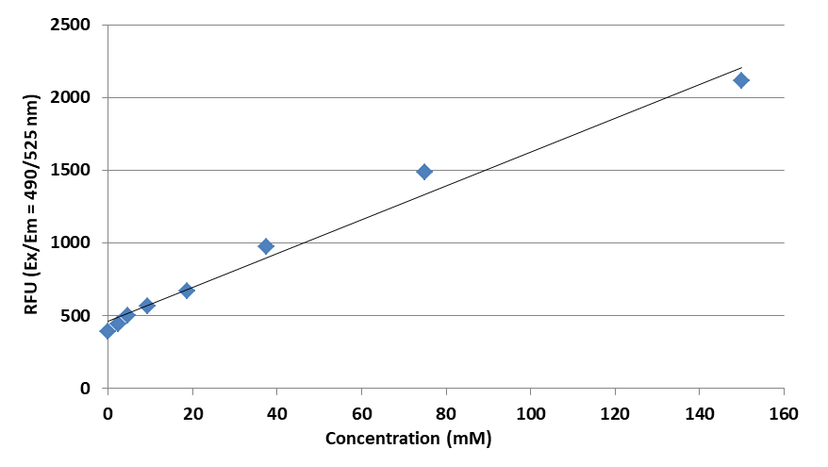
Lithium dose response was measured with Amplite® Fluorimetric Lithium Ion Kit in a 96-well solid black plate.
In recent years, fluorescent indicators have been researched for use in lithium quantitation. Benefits of fluorescent indicators include their commercial availability, detection in sub-millimolar concentration ranges, and rapid, easy, and effective mechanism. The largest challenge in creating fluorescent indicators specific to lithium ions is that they need to be able to compete with coordinating alkali ions, like sodium and potassium (Na+, K+), and alkaline earth metal ions, like calcium (Ca2+). Though various fluorescent indicators exist for the detection of other metallic ions, they have been less studied specifically in the capacity to identify lithium ions due to the lack of inherently biological relevance.
Ionophore-Based Sensors
Crown ethers are macrocyclic oligomers of ethylene oxide characterized by their selective binding abilities toward cationic and neutral species. In 1967, Charles Pederson was the first to discover an efficient strategy to prepare macrocyclic polyethers and subsequently study their binding properties towards metal cations. Today, crown ethers have been instrumental for the advancement of the field of supramolecular chemistry and have found widespread application, so much so that many types are commercially available. Crown ethers are able to selectively bind alkali and alkaline earth ions. The resulting complexes are soluble in non-polar solvents, thus acting as carriers of the charged species. They have been extensively used in phase transfer catalysis and ion extraction. Because crown ethers can generate stable supramolecular complexes with a wide variety of organic guests in aprotic solvents they have widely been adopted in ionophore technologies. These can easily form complexes with specific ions and facilitate their transport across cellular membranes.
In current research, ionophores with high affinities for lithium have been created; they can not only reliably and efficiently extract lithium ions from aqueous solution into organic solvents, but also show easy workability when incorporated into ion-selective membranes or electrodes. Ionophore-based sensors have been shown to possess high sensitivity, reproducibility, and high selectivity due to complementarity between the cations' electrostatic properties and those of the macrocyclic cavity. Applications of such portable, lithium ionophore sensors may allow for the accurate detection and monitoring of lithium ions in situ, in hospitals, remote locations, landfill sites or offshore.
Product Ordering Information
Table 1. Lithium Ion Detection Kits
Cat# ▲ ▼ | Product Name ▲ ▼ | Unit Size ▲ ▼ |
21351 | Amplite® Fluorimetric Lithium Ion Quantification Kit | 100 Tests |
21353 | Portelite™ Fluorimetric Lithium Ion Quantification Kit |
References
Optical lithium sensors
A Simple Assay for the Fluorometric Detection of Lithium Ions in Aqueous Solution
Paper-Based Device for the Facile Colorimetric Determination of Lithium Ions in Human Whole Blood
Lithium Ion Sensors
Fluorescence Sensors for Lithium Ions and Small Peptides
Tracking Lithium Ions via Widefield Fluorescence Microscopy for Battery Diagnostics
Direct synthetic routes to functionalised crown ethers
Determination of blood lithium-ion concentration using digital microfluidic whole-blood separation and preloaded paper sensors
The role of the size of aza-crown recognition moiety in azaphthalocyanine fluorescence sensors for alkali and alkaline earth metal cations
Lithium