A Practical Guide for Labeling Antibodies
Introduction
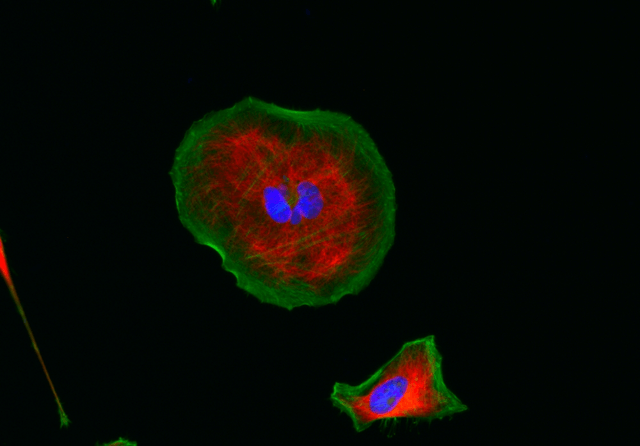
Example of fluorophore-labeled antibodies used to visualize cellular structure via direct detection. HeLa cells were stained with mouse anti-tubulin followed with iFluor® 555 goat anti-mouse IgG (H+L) (red); actin filaments were stained with Phalloidin-iFluor® 488 conjugate (green); and nuclei were stained with DAPI (blue).
Antibody Structure
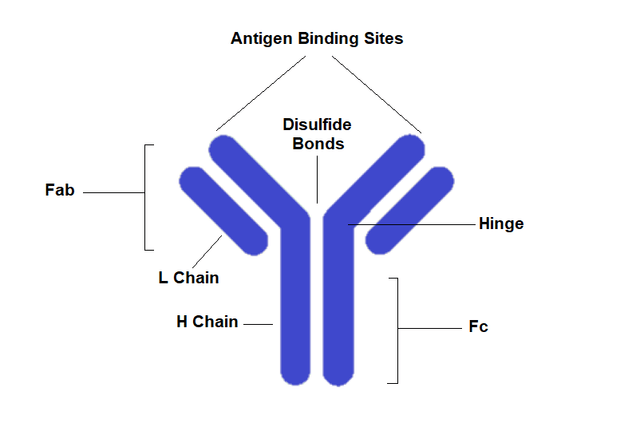
Simplified illustration of antibody structure. The highlighted areas include the fragment antigen binding region (Fab) at upper left, fragment crystallizable region (Fc) at lower right, the heavy chains (H chain) at the lower left, the light chains (L chain) at center left. At the very top are the antigen binding sites, being the two tips of the branching Y. In the center of the image is the hinge region, representing the central disulfide bonds of the antibody structure.
Types of Antibody Labeling and Their Uses
The 2 primary types of antibody labeling are amine-reactive and thiol-reactive labels, named for which area of the antibody is to be modified.
Amine-Reactive Dyes
Amine-reactive labels target primary amines (also called lysine groups) that are interspersed throughout the peptide chains of the antibody. This method is the most popular because of the prevalence of these (-NH2) groups in most antibodies, making it an effective default choice. The reaction is stable above a pH level of 7.5, the default concentration is a simple 1mg/mL, and the equipment requirements are minimal, making it an excellent choice for most laboratories. Reflective of this popularity, there are many amine-reactive fluorescent labels available commercially, with the most popular choice being succinimidyl esters (SE). Well-known examples include Thermo Fishers Alexa Fluor® and AAT Bioquests iFluor® SE dye series. This type of labeling is used for many biomolecules aside from antibodies, including peptides and nucleic acids, for applications ranging from fluorescence in situ hybridization (FISH) to immunohistochemistry (IHC). A potential drawback of this labeling method is a tendency towards poor shelf life, necessitating immediate use of the resulting conjugate. This method can be challenging to optimize, since under- or over-labeling the antibody will affect the performance of the resulting conjugate.
Thiol-Reactive Dyes
Thiol-reactive labeling targets the sulfhydryl bonds (-SH) on the outer portions of antibodies. This method is popular when conjugating larger molecules like phycoerythrin (PE), alkaline phosphatase (AP), or allophycocyanin (APC). AAT Bioquest has a guide available specifically for PE and APC conjugate applications, which have specific challenges. The appeal of this method for larger conjugates is that the antibody binding sites at the upper tips of each Y are left open. By not interfering with these binding sites, researchers can minimize the effect of labeling on the activity and viability of the antibody. Additionally, since these bonds only occur in specific locations, thiol-reactive labeling is an ideal choice if site-specific, controlled conjugation is required. There are multiple thiol-reactive labels available, with a popular variety being maleimides, used after the application of a reducing agent such as tris(2-carboxyethyl)phosphine (TCEP) or dithiothreitol (DTT). Specifically for large enzyme conjugation, techniques such as SMCC crosslinking are typically required. These compounds have a maleimide group on one end and an amine-reactive group such as an NHS ester on the other. Although effective, this method requires extensive biochemical background to optimize, and can have very low yields. Modern kits, such as the Buccutite™ series, bypass this requirement as well as attendant purification steps, allowing 100% recovery of the resulting conjugates. For a detailed example protocol for maleimide labeling, consult the Labeling Proteins with iFluor® Dye Maleimides application note.
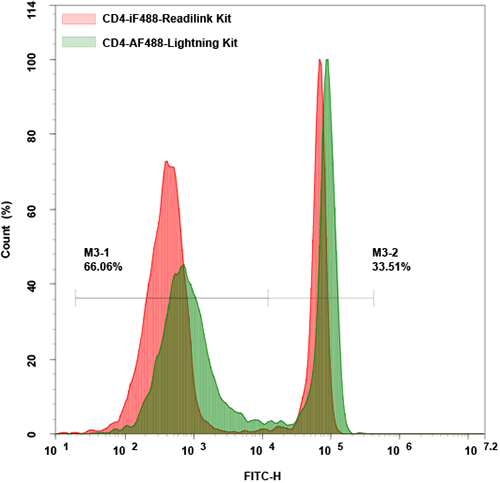
Flow cytometry analysis of human PBMC cells. Anti-human CD4 antibody prepared with ReadiLink™ Rapid iFluor488 Antibody Labeling Kit (Cat No. 1255) or Lightning-Link® Rapid Alexa Fluor ® 488. The fluorescence signal was monitored using ACEA NovoCyte flow cytometer in the FITC channel.
Antibody Labeling Kits
For smaller labs, employing an antibody-labeling kit is usually preferred, for reasons of efficiency. It is often useful to compare the performance of 2 or more kits before the final selection, as results can vary between cell lines and antibody types. (See Figure 2 for an example of a comparison)
Labeling Kit | CD4(SK3)- Conjugate | Stain Index |
ReadiLink™ Kit | CD4-iFluor® 488 Conjugate | 43 |
Lightning-Link® Kit | CD4-Alexa Fluor® 488 Conjugate | 26 |
Detection of Labeled Antibodies
The 2 major categories of antibody detection are either direct (chemically binding a fluorophore or other traceable label to a target antibody) or indirect (conjugating a directly-labeled secondary antibody to the target antibody).
For highly-expressed targets, direct detection is usually employed, since it is simple and efficient. For rarer targets, indirect detection is preferred due to the option of amplifying the signal with multiple labels per target. Tyramide signal amplification (TSA) is a popular option for improving low-abundance readings, recently superseded by Power Styramide™ signal amplification (PSA™). Indirect detection varies widely based on the downstream application, and developing optimized protocols, including all necessary positive and negative controls to verify specific binding, is a time-consuming process. Many researchers prefer to adapt known procedures, adjusting as necessary.
Direct Antibody Detection
Many techniques, including immunofluorescence and immunohistochemistry, use direct detection, which gives quick, effective visualization of most targets. The conjugated antibody provides a signal in reaction to stimulus (in the form of an added substrate or wavelength of light) commensurate with the amount of antibody present. Each antibody can only be linked with one reporter molecule, which makes quantification simple, but also means that the signal cannot be enhanced. For rare targets, this can mean that the lower signal can be difficult to detect.
Simplified illustration of direct vs. indirect antibody detection mechanisms. The target antibodies are each shown attached to the experimental surface (shown as a blue line) such as in common microplate assays such as ELISA. HRP has been chosen in this example as a common label. On the left is the direct detection mechanism, with an HRP-conjugated primary antibody reacting with a colorimetric substrate. On the right is the indirect detection mechanism. The target antibody has been bound by multiple HRP-conjugated secondary antibodies, each of which reacts with the colorimetric substrate, giving a more intense signal.
Indirect Antibody Detection
Indirect detection is an extension of direct detection, with secondary, tag-conjugated antibodies used in sequence with the primary target antibody. The general procedure is largely the same, with the protocols being determined by which portion of the primary antibody will be targeted (amine, thiol, or other type), and then the selection of the secondary antibody that will provide the experimental signal. Secondary antibody selection is a major component of successful protocols. Ideally, the secondary antibody will be highly specific to the target (minimizing general protein binding and the resulting undesirable background noise) and be able to bind at multiple points on the target (to amplify the signal via multiplicative conjugation). This signal amplification is crucial for highly-sensitive assays and very low-abundance targets. The secondary antibody chosen must also be conjugated to the correct reporter tag (enzyme, fluorophore, etc.) as needed by the experiment. One major advantage of indirect detection is the ability to conjugate multiple reporters to a single primary target via multiple secondary antibodies conjugated to different tags. The downstream application (Flow cytometry, western blot, etc., will determine the optimal tags chosen.
Simplified illustration of indirect detection and amplification, using PSA™ as an example. The primary antibody is shown at the bottom of each figure, colored in purple. The HRP-secondary conjugate (colored gold) is then bound to the primary target. HRP catalyzes the conversion of labeled inactive Styramide™ into highly-reactive Styramide™ radicals that covalently bind to tyrosine residues on and proximal to the enzyme site. The detectable signal is thus amplified exponentially.
Avoiding Common Difficulties and Challenges
Be mindful of downstream uses of the antibody. For amine-reactive direct labeling techniques, the TCEP reducing agent is incompatible with many assays, due to excessive background noise or other undesirable reactions. Use the ReadiUse™ TCEP removal solution or a similar alternative in order to eliminate residual TCEP in the reaction mixture.
Optimize antibody concentration. The antibody concentration recommended is at least 1mg/ml for most antibody labeling protocols, but can be modified as necessary if results are suboptimal. For experiments where the antibody availability is very low, using an antibody labeling kit that does not require a purification step (allowing 100% retrieval) is recommended. See this table for options.
Select buffers with care. Any buffer that contains amino acids, such as Tris buffer, will react with labels such as SE dyes. A very common mistake is not ensuring that buffers are free of BSA and gelatin. These proteins will also react with most labels, leading to significant loss of material. Consult provided protocols, but if no buffer is specified, the assumed default is typically a physiological buffer such as PBS or acetate. For buffer preparations and recipes, see Additional Resources below or use the AAT Bioquest Buffer Preparations and Recipes resource.
When selecting a secondary antibody, match the host species, class, and subclass of the primary antibody. In order to successfully bind to the target antibody, the secondary antibody must be raised against both the host and target species. For example, if the primary monoclonal antibody was raised in goats, then the ideal secondary antibody would be raised in a species other than goat, such as a rabbit anti-goat IgG. Polyclonal IgG antibodies will be recognized by anti-IgG antibody H+L (heavy and light chains). Consult our Secondary Antibodies selection page for more in-depth information.
Include all necessary controls. These include both positive and negative controls, with others as needed. For antibody labeling, in order to verify accurate, specific binding, and to minimize detection errors, there are multiple varieties. These include isotype controls, (primary antibodies included to confirm that the binding of primary antibodies is specific to the target of interest) unstained controls, (experimental sample without labeling, to determine base autofluorescence from the cells themselves) and antibody-isolate controls (to determine what degree of antibody binding is occurring with other proteins present in the sample). Others are often necessary in order to establish confidence in experimental results.
Additional Resources
AAT Bioquest Degree of Labeling Calculator
This is an open-access online tool for determining the number of dye molecules conjugated to a particular protein. The DOL is an important quantity when trying to optimize an experiment. Typically, too many dyes attached to a protein will result in a self-quenching effect, reducing the net fluorescence of the conjugate. On the other hand, insufficient labeling with too few dyes will also result in suboptimal fluorescence intensity. The DOL Calculator can be used to assess the optimal conjugation ratio.
A practical guide for use of PE and APC in flow cytometry
This AssayWise article provides a general overview of what phycoerythrin (PE) and allophycocyanine (APC) can provide in flow cytometry and FACS applications, and how to optimize their uses.
AAT Bioquest Buffer Preparations and Recipes
This is an open-access interactive resource of common buffers, cell media, and other solutions typically used in laboratories. The recipes are sorted by application, and include full instructions suitable for novice researchers.
On-Demand Webinar: Modern Methods for Fluorescent Antibody Labeling
This webinar is intended for researchers in both small- and large-scale laboratories, and covers common mechanisms and methods of bioconjugation, focusing on antibody fluorescent labeling, with an emphasis on the selection of appropriate techniques and materials.
References
- Alam, M. K., El-Sayed, A., Barreto, K., Bernhard, W., Fonge, H., & Geyer, C. R. (2019). Site-specific fluorescent labeling of antibodies and diabodies using SpyTag/SpyCatcher system for in vivo optical imaging. Molecular
- Kennedy, D., Cronin, U. P., Piterina, A., & Wilkinson, M. G. (2019). Heat and chemical treatments affect the viability, morphology, and physiology of Staphylococcus aureus and its subsequent antibody labeling for flow cytometric analysis. Applied and environmental microbiology, 85(17), e01006-19. https://doi.org/10.1128/AEM.01006-19
- Sun, W., Huo, Y., Mei, Y., Zhou, Q., Zhao, S., & Zhuang, M. (2020) Identification of a Small Probe That Can Be Conjugated to Proteins by Proximity Labeling. ACS Chemical Biology. 15 (1), 39-43 https://doi.org/10.1021/acschembio.9b00842
- Tertel, T., Bremer, M., Maire, C., Lamszus, K., Peine, S., Jawad, R., & Görgens, A. (2020). High?Resolution Imaging Flow Cytometry Reveals Impact of Incubation Temperature on Labeling of Extracellular Vesicles with Antibodies. Cytometry Part A. https://doi.org/10.1002/cyto.a.24034
- Yu S, He X, Drelich A, et al. (2019). A practical strategy for immunofluorescent detecting multiple targets in mouse tissues without restrictions on the host specious resources of the primary antibodies. Pathology, Research and Practice. 215(5):1049-1053. https://doi.org/10.1016/j.prp.2019.02.013