Cloning
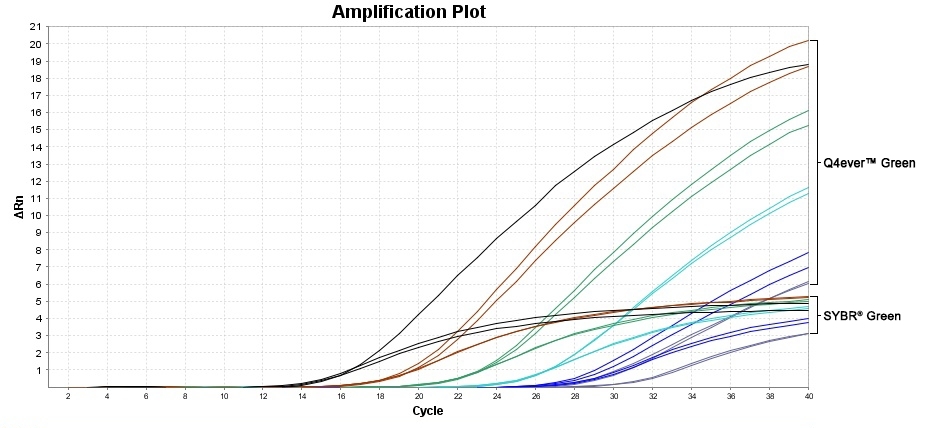
A comparison of the relative fluorescence signal from qPCR reactions performed with Q4ever™ Green and SYBR™ Green. Q4ever™ Green has much brighter signal than SYBR™ Green.
The procedure requires a vector, which is a circular DNA molecule that is used as a vehicle to facilitate the creation of recombinant DNA. The vector helps transport the target gene into a host cell and is generally a bacterium, though yeast and mammalian cells may also be used. In cloning, the foreign DNA fragments (also, the “inserts”) are first inserted into the vector and then reintroduced into a host for replication. As the host cells divide, they copy their own DNA along with the vectors, thereby replicating the insert.
Planning the Experiment and Vector
Fluorescence images of E. coli HST08 stained with Cell Meter™ Bacterial Viability Assay Kit. Live bacteria with intact cell membranes showed green fluorescence (Left), while 70% alcohol-killed dead bacteria (Right) with compromised membranes showed red fluorescence. Live and dead E.coli bacterial cells were also visualized in a mixed population (Middle).
Though molecular cloning can be performed through a number of different methods, each technique has similar key elements. An appropriate host organism must first be chosen, typically a non-pathogenic laboratory strain of Escherichia coli. Importantly, several strains are commercially available that have been genetically engineered for optimal performance in differing applications. An appropriate cloning vector must also be chosen. The selection is often a bacterial plasmid due to its simplicity, versatility, and ability to hold up to around 20,000 base pairs of foreign DNA. Outside of plasmids, other common examples of vectors include bacteriophages, cosmids, and artificial chromosomes. In general however, the vector should be selected, based on the specific host cell type used (e.g., mammalian or bacterial).
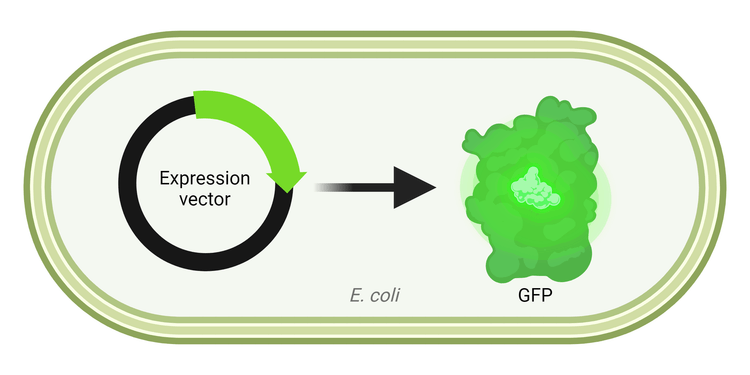
Illustration of an E. coli GFP expression vector permitting easy tracking of successfully-edited plasmids. Figure made in BioRender.
If desired the vector may include a protein tag, a small molecule that fuses to the insert. Protein tags allow for easy monitoring of protein localization of DNA downstream and some common examples include green fluorescent protein (GFP), luciferase, and glutathione-S-transferase (GST). Additionally, a genetic selectable marker may be introduced into a vector to confer a trait suitable for artificial selection. For instance, mammalian cells commonly utilize drug resistance genes as a selectable marker to detect antibiotic resistance. Lastly, the presence and length of the poly-adenylation signal on the vector must be considered. This is the portion of the vector located after the insert, which induces the termination of the messenger RNA (mRNA) transcript.
Preparation and Cloning
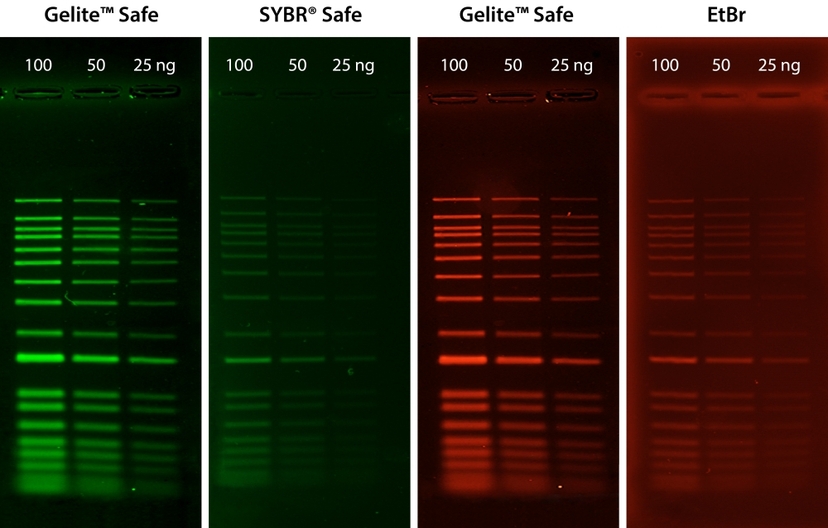
Comparison of DNA detection in 1% agarose gel in TBE buffer using Gelite™ Safe, EtBr, and SYBR® Safe. Two-fold serial dilutions of 1 kb DNA ladder were loaded in amounts of 100 ng, 50 ng, and 25 ng from left to right. Gels were stained for 60 minutes with Gelite™ Safe, EtBr, and SYBR® Safe according to the manufacturer's recommended concentrations and imaged using the ChemiDoc™ Imaging System (Bio-Rad®). Gels were illuminated using a 300 nm transilluminator fitted with a GelGreen filter.
Table 1. Non-toxic DNA stains for agarose gel electrophoresis
Product ▲ ▼ | Ex (nm) ▲ ▼ | Em (nm) ▲ ▼ | Unit Size ▲ ▼ | Cat No. ▲ ▼ |
Gelite™ Safe DNA Gel Stain *10,000X Water Solution* | 513 nm | 552 nm | 1 mL | 17702 |
Gelite™ Safe DNA Gel Stain *10,000X DMSO Solution* | 513 nm | 552 nm | 1 mL | 17706 |
Gelite™ Green Nucleic Acid Gel Staining Kit | 254 or 300 nm1 | Long path green filter2 | 1 Kit | 17589 |
Gelite™ Orange Nucleic Acid Gel Staining Kit | 254 or 300 nm1 | Long path green filter2 | 1 Kit | 17594 |
Helixyte™ Green Nucleic Acid Gel Stain *10,000X DMSO Solution* | 497 nm | 521 nm | 1 mL | 17590 |
Helixyte™ Green Nucleic Acid Gel Stain *10,000X DMSO Solution* | 497 nm | 521 nm | 100 µL | 17604 |
Helixyte™ Gold Nucleic Acid Gel Stain *10,000X DMSO Solution* | 496 nm | 539 nm | 1 mL | 17595 |
Once the vector is created, the insert must be prepared. An insert made of genomic DNA (gDNA) can be purified from any organism, though before cloning the DNA needs to be cut with a restriction enzyme or sheared to produce fragments of the target size. The insert may also be made from cDNA, obtained from mRNA by reverse transcription, or if preferred plasmid DNA that already contains the sequence of interest may be used. If using plasmid DNA, the target sequence should first be removed from the donor plasmid using appropriate restriction enzymes, then size-selected before cloning.
Datasets: |
PCR products can also be used for inserts, and blunt-ended products can either be used directly or cut with restriction enzymes. Lastly, synthetic DNA may be preferred, created in vitro. Since synthetic DNA utilizes a pre-existing DNA template, this insert type allows the highest level of flexibility in manipulation. Note: Like inserts from PCR products, sequencing of the cloned insert should be performed after preparation since mutations can be common during DNA synthesis.
After both the vector and insert are prepared, they are mixed together and a DNA ligation reaction is performed using a select enzyme. Next, the vectors containing the insert are introduced into the host system, most often by means of chemical transformation. This is performed by pretreating host cells with various chemicals under cold conditions, followed by a short heat shock. Alternatively, electroporation may be used where cells are subject to a brief electric shock that generates small pores into the cell surface, thus allowing external DNA to enter. Chemical transformation, however, remains the method of choice due to its increased simplicity and superior post-procedure cell viability.
Once the vector is fully integrated into the host cells, the cells are cultured appropriately with a selection agent which is usually an antibiotic. After culture, cells can be isolated, expanded through PCR or restriction digest, and then analyzed by DNA sequencing. Note: DNA sequencing is especially vital in some instances to ensure that the insert is present and correctly inserted. Finally, the clone carrying the recombinant DNA is expanded in culture to amplify the product. The amplified vector sequence can then be extracted and purified using appropriate protocols or commercial kits for further use.
Methods of Molecular Cloning
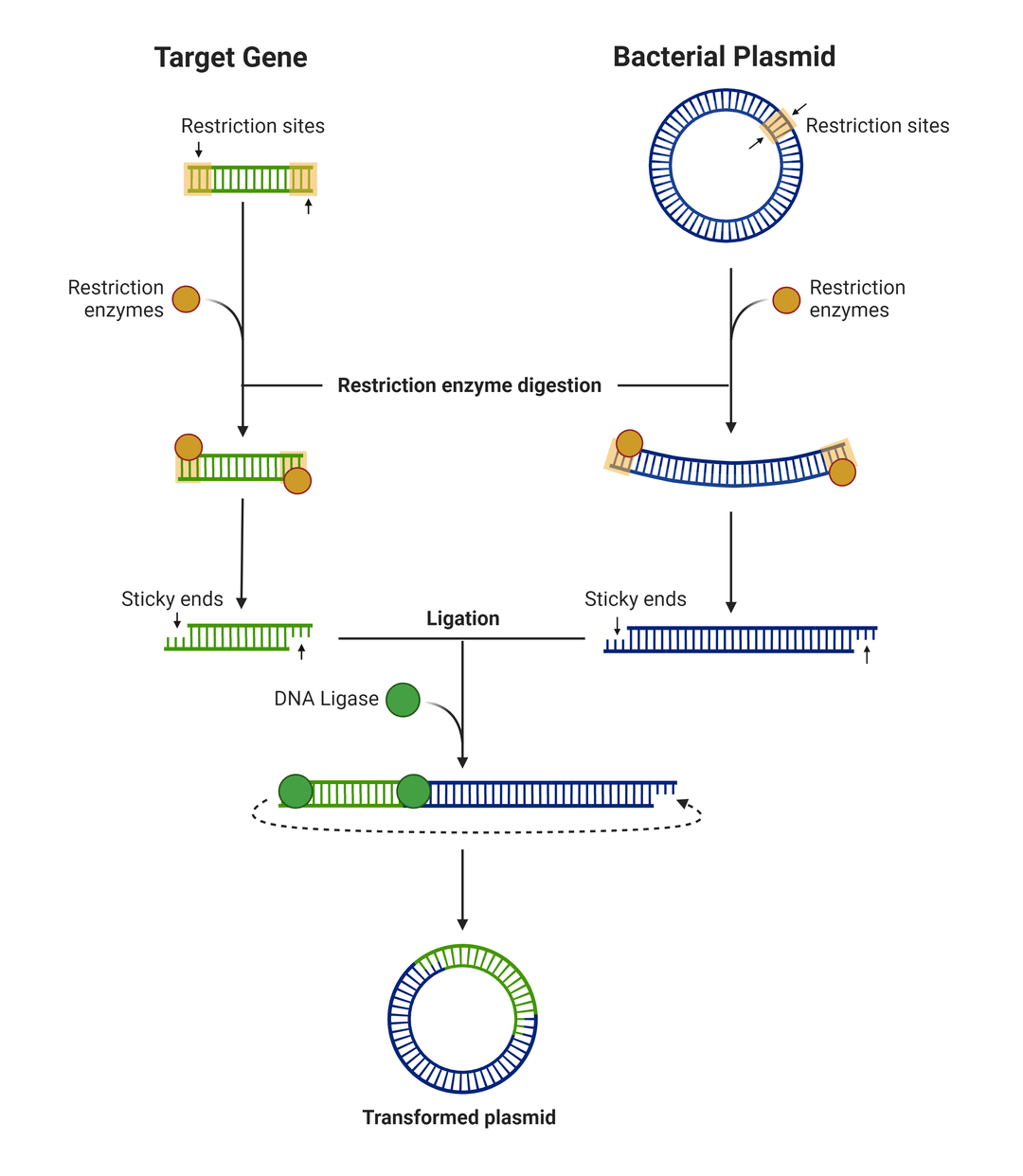
Simplified process of the restriction enzyme cloning process, in which the gene of interest is inserted within a plasmid using restriction enzymes. Figure made in BioRender.
Due to the short length of fragments produced, adding restriction sites into the DNA during PCR amplification is also possible. As restriction enzyme cloning does not require polymerase to create a fragment, there is less risk of introducing a mutation into the DNA. Unfortunately the ligation step can be tricky to accomplish and it is difficult to get successful ligation of numerous fragments in tandem. Restriction enzyme cloning requires convenient, specific, unique sites within the DNA sequence for appropriate fragmentation. Additionally, this method can produce extraneous sequences that present as short scars within the cloned DNA.
Molecular cloning through PCR involves the direct ligation of a PCR-generated DNA fragment without using restriction enzymes or exogenous ligases. PCR cloning is fast, versatile, and can produce millions of clones from a single nucleotide sequence. The most commonly used PCR cloning method is toposiomerase based cloning (TOPO or TA cloning) which relies on the hybridization of the complementary base pairs adenine (A) and thymine (T). TA cloning relies on three major components: the adenine residue overhang that is added by Taq polymerase at the 3' ends of PCR products, the complementary thymine that comes from the 5' ends of pre-cut linear vectors, and topoisomerase which ligases the residues together. Careful selection of TA cloning vectors can save time in experimentation, and high-quality cloning reagents can help ensure the creation of consistent, identical clones. Some of the major disadvantages of PCR cloning lie in that it can easily introduce mutations and extraneous sequences into cloned products.
Ligation independent cloning (LIC), also termed in-fusion cloning, is another method that does not not require site-specific recombination or a ligation step. In this technique, T4 DNA polymerase chews back the 3' end of dsDNA to create 5' overhangs, and then complementary strands between the vector and insert are annealed together. In-fusion cloning is easily accomplished by using modified primers during PCR amplification. Though no scars are created in the resulting clone, it will initially have four single stranded DNA (ssDNA) nicks that are later repaired by the host organism. This method, however, tends to be expensive and since PCR is required, mutations might be present. Additionally, in-fusion cloning is highly sensitive to timing of each step, so quantitation and sequences that are prone to a secondary structure may be more difficult to clone with this technology.
Recombinational cloning, also termed gateway cloning, is a universal technique that uses site-specific DNA recombinases. Recombinases are enzymes capable of swapping pieces of DNA between two molecules that both contain the recombination sites. Gateway cloning is based on the highly specific integration and excision reactions of bacteriophage λ into and out of bacteria from the E. coli genome. In the process, first the appropriate recombination sites are inserted by PCR on either side of the insert. Then the product is recombined with a donor vector to create an entry clone, and this entry clone is recombined again with a destination vector to create the final clone. Recombinational cloning offers high throughput capabilities, does not require repeat sequencing verification, and can combine multiple fragments in one reaction. Sequence scars, however, are inevitable in this procedure, and the propagation of vectors should be carefully considered. Another con of this method lies in that steps, reagents, and enzymes can be relatively expensive.
Type IIS method of molecular cloning, also termed Golden Gate cloning, takes advantage of the unique properties of type IIS restriction endonucleases that bind at a specific sequence and cut exactly 5 bps away. Essentially, the enzyme cleaves its own binding site and leaves behind sticky overhangs. As type IIS restriction endonuclease cuts distal to its recognition site, customizable overhangs are possible. In the process, the entire cloning step including digestion and ligation can be carried out in one reaction, and no undesired nicks or scars are left in the recombinant DNA. Though this method is fast, seamless, and produces no background colonies, application should only be considered for certain types of cloning projects and requires acute, careful planning.
Simplified steps of the process of isothermal cloning, which permits multiple insertions of dsDNA fragments, as long as each has matching overhangs. Figure made in BioRender.
Isothermal cloning, also known as the Gibson assembly method, allows for a sequence-independant and scar-free insertion of 1-10 DNA fragments into a vector. Similar to in-fusion cloning, the Gibson assembly method relies on the addition of regions of homology at each end of the targeted inserts. This method relies on the interaction of three players to generate recombinant DNA: DNA ligase chews back 5' ends to create 3' compatible overhangs on the sequences, while DNA polymerase fills in gaps in the annealed fragments, and DNA ligase seals the nicks in the assembled DNA clones. In the Gibson assembly method, DNA fragments with 20-40 bp homology at their ends can be ligated together in one reaction, without using restriction enzymes. Any dsDNA fragments can be used in this technique, so if properly designed, any insert with appropriate overhangs can be efficiently ligated into any vector backbone.
FAQs: | Datasets: |
The last common method of molecular cloning involves a yeast-mediated reaction. Yeast-mediated cloning is very similar to Gibson cloning, but instead utilizes the powerful recombination abilities of yeast. Yeast-mediated cloning is able to fuse together two or more fragments of dsDNA that have at least 30 bp of overlapping homology. Compared to other methods, much larger final constructs can be generated, that usually range in size up to 100 kbp. Yeast-mediated reactions provide the ability for oligonucleotide stitching, which occurs when pieces of DNA that share no end homology can still fuse together in a seamless manner. Though simple and relatively inexpensive, a major disadvantage of this technique is that the setup requires lengthy steps including growing, transforming and purifying DNA from yeast.
Product Ordering Information
Table 2. Nucleic acid stains for agarose and polyacrylamide gel electrophoresis
Product ▲ ▼ | Ex (nm)¹ ▲ ▼ | Filter² ▲ ▼ | Unit Size ▲ ▼ | Cat No. ▲ ▼ |
Helixyte™ Green Nucleic Acid Gel Stain *10,000X DMSO Solution* | 254 mn | Long path green filter | 1 mL | 17590 |
Helixyte™ Green Nucleic Acid Gel Stain *10,000X DMSO Solution* | 254 mn | Long path green filter | 100 µL | 17604 |
Helixyte™ Gold Nucleic Acid Gel Stain *10,000X DMSO Solution* | 254 mn | Long path green filter | 1 mL | 17595 |
Gelite™ Green Nucleic Acid Gel Staining Kit | 254 nm or 300 nm | Long path green filter | 1 Kit | 17589 |
Gelite™ Orange Nucleic Acid Gel Staining Kit | 254 nm or 300 nm | Long path green filter | 1 Kit | 17594 |
Gelite™ Safe DNA Gel Stain *10,000X Water Solution* | 254 nm, 300 nm or 520 nm | Ethidium Bromide, Gel Star, Gel Green, Gel Red and SYBR filters | 100 µL | 17700 |
Gelite™ Safe DNA Gel Stain *10,000X Water Solution* | 254 nm, 300 nm or 520 nm | Ethidium Bromide, Gel Star, Gel Green, Gel Red and SYBR filters | 500 µL | 17701 |
Gelite™ Safe DNA Gel Stain *10,000X Water Solution* | 254 nm, 300 nm or 520 nm | Ethidium Bromide, Gel Star, Gel Green, Gel Red and SYBR filters | 1 mL | 17702 |
Gelite™ Safe DNA Gel Stain *10,000X Water Solution* | 254 nm, 300 nm or 520 nm | Ethidium Bromide, Gel Star, Gel Green, Gel Red and SYBR filters | 10 mL | 17703 |
Gelite™ Safe DNA Gel Stain *10,000X DMSO Solution* | 254 nm, 300 nm or 520 nm | Ethidium Bromide, Gel Star, Gel Green, Gel Red and SYBR filters | 100 µL | 17704 |