A Practical Overview of qPCR
Abstract
The polymerase chain reaction (PCR) is one of the central procedures in multiple areas of research science and has facilitated the expansion and improvement of many applications, including GMO detection or in assessing the presence or absence of a DNA target. For some research applications, all that is necessary is to know whether or not a target of interest is present or absent in a sample, with sensitivity being the primary concern. However, qualitative analysis is necessary to determine how much of the target of interest is in the sample. Real-time qualitative PCR, abbreviated as qPCR, permits more information (such as relative or absolute starting measurements of sample DNA) to be gleaned from small samples. Keeping all procedural steps within a single tube or well improves time efficiency and minimizes the potential for sample contamination. This piece will be an overview of qPCR principles, materials, basic procedures, and common difficulties and challenges.
Introduction
During PCR amplification, each strand of dsDNA is theoretically doubled, so 1-2, 2-4, 4-8, and such. This rarely, if ever, happens, and the efficiency (Qn) is highly unlikely ever to be 100.
Q0 x 2n = Qn
In the equation above, Q0 is the starting quantity, Qn is the ending quantity, and n is the number of cycles.
For standard PCR, all nucleic acid detection is done at the very end of the entire set of 40 cycles. Real-time PCR means nucleic acid detection is done at the end of every cycle. This is done by incorporating the fluorescent marker into the nucleic acid at the very beginning of the procedure, and the fluorescence can be measured as the process runs. This permits quantifying the amount of dsDNA in the original sample, leading to the usual acronym qPCR.
The two kinds of quantification are absolute and relative. In absolute, by comparing our unknown sample to the standard/control curve, we can calculate the starting quantity using a calculated log value known as the cycle of quantitation (Cq). Relative quantification compares the base versus the treated sample, and the difference between them is calculated. The differences are primarily in sample amounts. In relative quantification, no control curve is required.
In qPCR, adding a fluorimeter and fluorescent labels to the base PCR protocol permits a vast increase in possible research applications. By integrating a fluorescent marker into the denaturation, annealing, and elongation steps, qPCR can give information at every stage of nucleic acid amplification instead of simply being a preliminary step for eventual sample analysis. These real-time readings are not only helpful information in their own right but permit the computation of the amount of original genetic material (the number of copies of the amplified target sequence), as well as the Cq value at the end of each cycle step.
There are two major subtypes of qPCR, which primarily differ by the type of marker used for fluorescent measurement.
Types of qPCR
What type of qPCR best suits an individual experiment depends on downstream applications and the acceptable costs in materials and time. As mentioned previously, there are two main types: dye-based (SYBR® Green intercalating dye and otherwise) or probe-based (with hydrolysis probes like TaqMan® being the most frequently used).
Dye-based qPCR
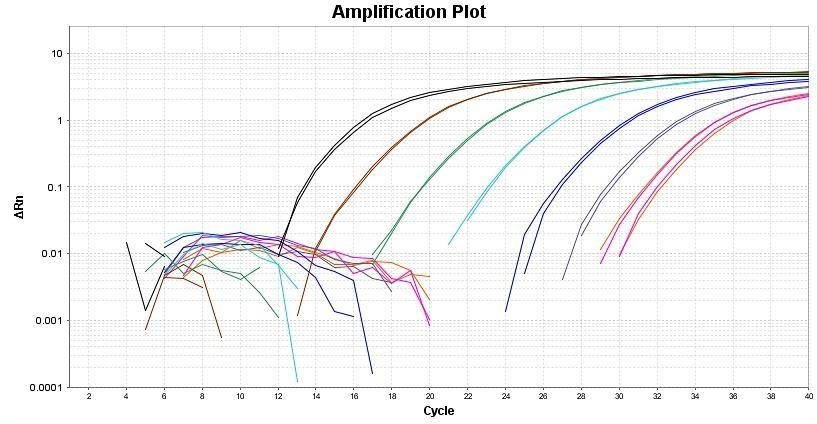
Dye-based qPCR is the simpler option of the two and only requires two sequence-specific DNA primers and a DNA-intercalating dye to generate a signal. Compared to microarrays, dye-based qPCR is more sensitive at detecting modest changes in expression levels, making it well-suited for investigating small subsets of genes. It is the most common choice for gene expression validation and DNA quantitation. The fluorescence generated is non-specific, so all the dsDNA present in the sample will generate a signal. This includes non-template amplification (NTC) and secondary structures like primer-dimers.
Dye-based qPCR requires a post-procedure step of a melt-curve analysis to assess the amplification reaction's degree of specificity. It also has the fastest 5′ to 3′ exonuclease activity so that the cycles can be run slightly faster than other qPCR options.
SYBR® Green is the most common DNA-binding dye for measuring PCR amplification, but many alternatives are available, such as Helixyte™ Green, Q4ever™ Green, and EvaRuby™ Dye. All of these dyes bind non-specifically to double-stranded DNA (dsDNA). These intercalating dyes provide a dependable signal, although they require careful handling.
Helixyte™ Green (equivalent to SYBR® Green) exhibits minimal fluorescence when it is free in solution. The fluorescence of the dye increases up to 1,000-fold after binding to DNA. More importantly, the fluorescence output is proportional to the amount of dsDNA present and increases as more PCR product is produced. The fluorescence signal increases exponentially with target amplification until the DNA primers are depleted.
Probe-based qPCR
For probe-based qPCR, the probe oligonucleotide markers are designed to be specific to the target of interest. The most common type of probe is the hydrolysis probe. The probe is broken during the annealing step so that it will fluoresce. Probe-based detection supports multiplexing since different probes (detected in other channels) can be combined to give more information within a single experiment.
A short 30-40 base-pair sequence-specific oligonucleotide is used, which includes a 5' fluorescent reporter and a 3' quencher. The reporter and quencher work in tandem. When broken (after the binding to dsDNA during annealing and subsequent breakage during the extension phase), the fluorescent reporter can give a signal without the quencher. The FRET-labeled probe gives sensitive, specific results, but developing specific probes can be time-consuming.
Probe-based qPCR is a favored method for measuring transcript abundance and is most frequently used in diagnostic assays. It is one of the most sensitive detection methods that provide an accurate and reproducible analysis. In probe-based qPCR, fluorescently-labeled target-specific probes are used to measure DNA amplification in real-time, offering the least background fluorescence compared to other (dye-based) chemistries. This method benefits from extreme specificity and allows the end-user to multiplex targets in a single reaction. The number is typically limited by the number of light channels found in the real-time PCR instrument. Several platforms are available that use probe-based chemistry to quantitate transcript abundance. Hydrolysis probes, such as TaqMan® probes and Molecular Beacons, are the most widely used.
Basic Materials, Procedures, and Sample Protocol
A qPCR experiment requires multiple components to run successfully. Mixed thoroughly (to ensure well-to-well or tube-to-tube consistency), the reaction buffer will include the DNA template, primers, and probes (if using), as well as the titular polymerase enzyme, magnesium or other cation, and dNTPs. It is incredibly time-consuming to optimize a qPCR procedure correctly, so using commercially-available master mixes has become common. These master mixes include validated balances of all necessary components except for the template and primers. Some will consist of an intercalating dye for dye-based qPCR.
Given this ever-increasing popularity, the following explanation will include master mixes. Adjusting these mixes is entirely possible, as is assembling an assay mixture from scratch, but it is a more advanced proposition that may be inaccessible for the beginning researcher.
Essential Materials
Successful DNA amplification requires concise control of temperature changes (using a well-maintained instrument) and quality reagents: master mix, primers, template, and lab-grade pure water.
- Master mix: A combination of deoxyribose nucleotide triphosphates (dNTPs), buffers, and DNA polymerase, plus any extra components like magnesium or manganese (a master mix is sometimes referred to as a supermix, which usually only has the dNTPs, buffer, and enzymes). Commercially available master mixes vary in performance, but for unusual requirements, a modified DNA polymerase sometimes gives better and more stable results.
- Primers: Short, forward, and reverse, usually only a handful of base pairs. These act as an initiation site for amplification to take place. Specificity is the highest priority, with length and other aspects as relevant. There will usually be both ‘upstream’ and ‘downstream’ primers relative to the template, with upstream being towards the 5’ end of the strand and downstream towards the 3’ end.
- Template: This is the actual nucleic acid of interest to the procedure. Its composition, length, and purity all can affect the amplification efficiency. An ideal length is usually ~100 base pairs and is typically one of 4 types: genomic, plasmid, viral, or cDNA.
- Water: Nuclease-free and lab-grade pure to avoid degradation of the starting template. This is particularly essential for assays using rare or irreplaceable samples, such as in forensics applications, which may already have some degree of damage.
Material Selection and Issues
The concentrations of materials (aside from premade kits) greatly impact qPCR results, and the ideal balance must be empirically tested. There are some standard ranges that are the most typically chosen and lend themselves well to reproducible results and are a helpful place to begin testing.
Master mix
Probe and dye mixes are similar in that they include everything needed to amplify a single target. Mastermix selection depends on ingredients but should be tested prior to use with any rare or irreplaceable samples since efficiency has a wide variance. Dye-based qPCR typically includes the intercalating dye into this mix at optimal concentration. Probe mixes do not include the fluorescent marker and can be adapted based on marker choice.
Tip
Normalize assay signal with a passive reference dye (such as ROX or higher-performing alternatives like 6-ROXtra™). This helps with the signal variance between wells due to the stationary light source in many instruments. Not all instruments will require a reference dye, but many will so include it as necessary based on your experimental setup. Many universal master mixes will consist of one of these passive reference dyes at differing concentration levels.
When selecting a premade master mix, compare the performances of the available options that contain the materials necessary for the experiment since there is a broad spectrum of performance and dynamic range exacerbated by sample and instrument type.
Examples of Mastermix Comparative Testing of PCR Efficiency
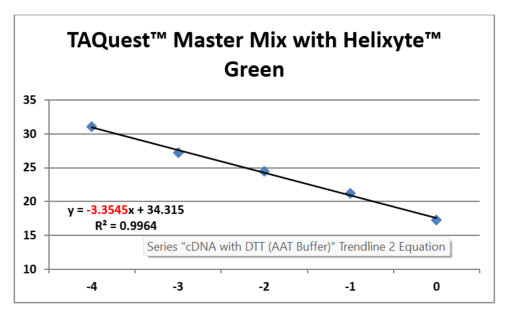
By running serial dilutions of the sample DNA concentration, we can determine the dynamic range, efficiency, and specificity of the qPCR assay. Dilutions are usually done by factors of 10 but may be done using alternative factors if required or desired. Once the amplifications have been run for each starting quantity, the Ct vs. log of the starting quantity can be assessed, with the slope determining the efficiency (E).
E = 10-1/slope
%E as (E-1) x 100
The percent efficiency should be as close to 100% as possible, which equates to a slope value of -3.32. A percent efficiency in the 90-110% range is usually considered optimal. An assay with 100% efficiency is rare and would represent a perfect doubling of the starting target per cycle. Low efficiency is generally caused by poor primer design, expired or low-quality reagents, or incorrect annealing temperature.
Tip
If efficiency is very high (well above 110%), there is likely a primer dimer, and off-target products are present. Assess the lowest concentration point on the dilution assay curve to see if that is changing the slope unduly, at the cost of a smaller dynamic range. Alternatively, check the melt curve for insight into any off-target products that may be present.
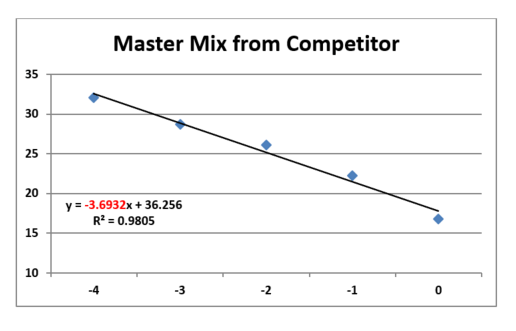
The y-intercept is the limit of detection (LOD) of the procedure. Although a qPCR assay could theoretically be sensitive enough to detect a single copy of the target, realistically, 2-10 copies are considered reliable.
R2 is the best-fit line of the ideal vs. the actual observed plotted line and is referred to either as the correlation coefficient or the coefficient of determination.1 An R2 value of 0.98 or above is considered acceptable: the higher, the better. It measures the linearity of the relationship between two variables. Although, in theory, the perfect R2 = 1, in practice, 0.99 and above is more realistic.
Variances usually occur at each end of the dilution range and may be omitted at the cost of a smaller dynamic range. If R2 is too low, we recommend redesigning the assay with different primers.
For the two example graphs, TAQuest™ Master Mix with Helixyte™ Green and Master Mix from Competitor, both R2 values are within the acceptable range, with the first TAQuest™ master mix showing a higher (0.9964) and more desirable value than the other (0.9805). Additionally, the second mix shows a slightly steeper slope (associated with over 100% efficiency, suggesting off-target amplification) than the first mix.
When selecting a mix, researchers may need to assess whether accuracy or efficiency is a higher priority and to what extent.
Primers
Primers are short single-stranded stretches of nucleic acids. They are the core concern for any procedure, and their specificity and efficacy are the most common stumbling block during optimization and validation of the assay. Given well-chosen primers, nearly all other aspects of the assay can be adjusted around them. A qPCR procedure will include both ‘upstream’ and ‘downstream’ primers, designed to match each end of the targeted DNA sequence (the template). By beginning the DNA synthesis at these endpoints, off-target amplification is minimized.
Assay results are highly dependent on primer sequences, so these sequences must be included along with the associated data in any resultant publications, per MIQE guidelines.2 Primers that are contaminated, truncated, or have incorrectly chosen bases, can destroy qPCR accuracy.
Tip
Primer pairs should not include complementary sequences/regions. If there is 3’ complementarity, it is likely to cause primer-dimer formation.
Generally, primer lengths of between 18-24 base pairs, beginning and ending with at least one G-C pair and containing approximately 40-60% G-C content, give good results. Most available primers will have a Tm of 50-60 °C.
When selecting multiple primers to be used in combination within a single assay (as in multiplex qPCR), ensure that the melting temperature (Tm) is within 5 °C. Mismatched Tm will cause asynchronous dsDNA amplification since they will dissociate at different stages of the thermal cycles.
Template
The template is the piece of DNA that is to be amplified. Ideally, it should be highly pure and of high quality. There are many sample sources where the genetic material may have been gleaned, with qPCR being useful for nearly any type.
Tip
If the genetic material is viral or a plasmid, then a much smaller quantity is needed, often with a final concentration of 1 pg-1 ng being the typical range. For cDNA or genomic DNA, concentrations are typically from 1 ng-1 µg.
The proportion of G-C pairs in a template can be an issue since their stronger bonds can give rise to primer-dimers, hairpins, and other secondary structures, which can skew assay signals. Although some optimization steps can help with these issues, in general, the development of secondary structures should be avoided if possible.
The template length must be considered when choosing a supermix since longer lengths typically require a polymerase with greater processivity. Polymerase attaches to a small number of bases and then disengages. The processivity measures how many bases that polymerase will process before it unlatches from the DNA strand.
Tip
In terms of efficiency and time at the bench, if the sample template is long, a polymerase with higher processivity should be chosen, so that cycle time doesn’t need to be extended.
Additionally, if there is a limited amount of the sample template, researchers may opt to choose a hot-start qPCR procedure (which begins the process at a slightly higher temperature) to minimize other enzyme activities and assist with specific amplification. Most, if not all, instruments will have this functionality.
Water
Lab-grade, enzyme-free pure water is a common requirement for many lab procedures, but it is of particular importance for qPCR since nucleases like RNAse and DNAse can degrade and corrupt samples quickly.
Table 1. TAQuest™ qPCR Master Mixes.
Product ▲ ▼ | Reference Dye ▲ ▼ | Unit Size ▲ ▼ | Cat No. ▲ ▼ |
TAQuest™ qPCR Master Mix with Helixyte™ Green | No Rox | 1 mL | 17270 |
TAQuest™ qPCR Master Mix with Helixyte™ Green | No Rox | 5 mL | 17271 |
TAQuest™ qPCR Master Mix with Helixyte™ Green | Low Rox | 1 mL | 17272 |
TAQuest™ qPCR Master Mix with Helixyte™ Green | Low Rox | 5 mL | 17273 |
TAQuest™ qPCR Master Mix with Helixyte™ Green | High Rox | 1 mL | 17274 |
TAQuest™ qPCR Master Mix with Helixyte™ Green | High Rox | 5 mL | 17275 |
TAQuest™ FAST qPCR Master Mix with Helixyte™ Green | No Rox | 1 mL | 17276 |
TAQuest™ FAST qPCR Master Mix with Helixyte™ Green | No Rox | 5 mL | 17277 |
TAQuest™ FAST qPCR Master Mix with Helixyte™ Green | Low Rox | 1 mL | 17278 |
TAQuest™ FAST qPCR Master Mix with Helixyte™ Green | Low Rox | 5 mL | 17279 |
Other consumables associated with qPCR are the sample holders to insert the materials into the experimental instrument and the pipette tips for transferring the samples. Depending on which instrument is used, tubes, tube strips, plates (deep well or microtiter), and their associated seals are the most common options. For selecting these materials, instrument compatibility and quality are the central concerns. The original suppliers/manufacturers of PCR instruments will list what consumable formats are compatible, and there are also online tools available that collate information for researchers.
The bare essentials of any real-time PCR instrument are a thermal cycler and an optical detector. There are a vast number of instruments available, with many lists available comparing them based on the intended use. Although a full exploration of every factor of thermocycler instrument selection is beyond the scope of this introductory piece, significant criteria include:
- Which imaging channels are necessary and how many (to facilitate which dyes your lab uses and how many will be used in combination)
- Adaptability if the custom experimental setup is desired (not required if ready-to-use kits will be the default)
- The intended volume and speed of the assay requirements (small-scale, HTS, or both)
Basic qPCR Procedure and Example Protocol
The actual thermal cycling procedure is relatively simple. The timing and temperatures for an assay are unlikely to need much adjustment.
To begin the amplification process, cDNA is heated up to 95 °C. This is the denaturing step since the strands will break apart into two single-stranded strands (complimentary). Annealing is done by dropping it to 48-72 °C (55-65 °C is most common). Although the annealing temperature varies, it is usually set at approximately 3-6 °C below the primer Tm. The extension is generally done at a similar or slightly higher temperature (68-72 °C) than annealing. This will permit the incorporation of the free-floating dNTPs, starting at the primer sequences, to assemble two new complete double-stranded DNA molecules.
Table 2. Overview of the basic steps in the qPCR cycling reaction
Step ▲ ▼ | Temperature ▲ ▼ | Time ▲ ▼ | Process ▲ ▼ |
Denaturation | 95°C | ∼20 to 30 seconds | Double-stranded DNA (dsDNA) template is heated to high temperature. This disrupts the hydrogen bonds between the complementary base pairs causing dsDNA to separate into single-stranded DNA (ssDNA).
|
Primer Annealing | 48 to 72°C | ∼20 to 40 seconds | After denaturation, the reaction temperature is lowered to ∼48 to 72°C. This promotes the binding of forward and reverse primers to each of the ssDNA templates and the subsequent binding of DNA polymerases to the primer-template hybrid.
|
Extension | 68 to 72°C | ∼1 to 2 minutes | After annealing, the reaction temperature is raised to ∼68 to 72°C. This enables DNA polymerase to extend the primers, synthesizing new DNA strands complementary to the ssDNA template in the 5’ to 3’ direction. |
These denaturing, annealing and extension steps are typically repeated approximately 40 times, with each iteration referred to as a cycle. Standard-base amplicons with approximately 100 or fewer base pairs will require a shorter cycle time. Many processes include the 3-step protocol, with the annealing step including an extension temperature of approximately 72 °C. These are ideal for longer amplicons (>150 bp), complex or unknown samples, or low-efficiency amplification. For some very short amplicons or well-validated reagents, the extension step can be shortened or omitted entirely.
Tip
Remember to have the plate be read at the end of the extension step, not the annealing step if you have made manual adjustments to the cycle that your instruments’ software does not automatically account for.
For a more thorough step-by-step explanation of PCR in general, consult ‘A Practical Guide for the Detection and Analysis of PCR Products'
Example Protocol
Note: This is a simple sample protocol, and while helpful as a guideline or a starting point, it should be adjusted or changed entirely based on your experimental requirements and optimization results.
The materials used are linked if more information is desired, and their requirements will be similar to others available on the market.
- Thaw the TAQuest™ qPCR Master Mix (select the correct level of included passive reference dye if any is necessary) at room temperature, and vortex thoroughly before use.
- Prepare one of the following reaction mixes as indicated below, selecting which one is applicable to the type of procedure being run.
- Carefully mix the regents with a gentle vortex followed by a brief centrifuge.
Note: Even if volumes are small, always balance the centrifuge according to best practices for safety. - Set up the plate, tubes, or strips in your qPCR instrument and run as indicated with the thermal cycling parameters in the bottom table.
Table 3. Components for Dye-Based qPCR Procedure
Components ▲ ▼ | Final Concentration ▲ ▼ |
TAQuest™ qPCR Master Mix with Helixyte™ Green | 1X |
Upstream primer, 10 µM | 0.1-1.0 µM |
Downstream primer, 10 µM | 0.1-1.0 µM |
DNA template | Optimized conc. |
Nuclease-Free Water | Depends on well/tube volume |
Table 4. Components for Probe-Based qPCR Procedure
Components ▲ ▼ | Final Concentration ▲ ▼ |
TAQuest™ qPCR Master Mix for TaqMan Probes | 1X |
Upstream primer, 10 µM | 0.1-1.0 µM |
Downstream primer, 10 µM | 0.1-1.0 µM |
TaqMan Probes, 10 µM | 100-250 nM |
DNA template | Optimized conc. |
Nuclease-Free Water | Depends on well/tube volume |
Table 5. Basic thermal cycling parameters:
Parameter | Polymerase Activation | PCR (40 cycles) | ||
Hold | Denature | Anneal | Extend | |
Temperature | 95 °C | 95 °C | 55-65 °C | 68-72 °C |
Time (m:ss) | 0:20 | 0:30 | 1:00 | 1:00 |
Instrument Readouts Associated With Real-Time Amplification
Real-time data is displayed with an amplification curve, taken by measurements at the end of each of the 40 amplification cycles. It always begins low and will grow quickly.
The three segments of the amplification curve will be the baseline phase, the exponential phase (made of the take-off and linear stages), and the plateau phase. After all 40 cycles are done, a melt curve is run if necessary (usually between 65-95 °C).
- Baseline: amplification has barely begun at this point, shown by a flat line, since the fluorescence signal is too low to be detected, even though there will be an increase in PCR product.
- Take-Off (usually around cycle 10 or so): There is an abundance of PCR reagents at this stage, marked by the exponential increase in amplification and commensurate signal. The threshold will be set in this region of the amplification curve, and it represents the best amplification efficiency and the best precision.
- Linear: Amplification decreases (usually because freely available dNTPs are diminished).
- Plateau: Amplification flattens, PCR reagents are depleted, and along with the self-annealing of PCR products, will show minimal or no additional amplification. This area also represents the lowest degree of specificity of the amplification.
There will be one curve for each labeled target. A single threshold line will be used for each of them, marking the point at which the detected fluorescent signal positively changes from the background signal. This will be the Cq point for each curve.
There are very few circumstances in which researchers might elect to run fewer than 40 cycles for a qPCR procedure. It is also unusual for more than 40 cycles to be necessary. If the amplification curve displays all expected phases a bit early, researchers may decide to remove the extras in the interest of time. That said, this reduction won't necessarily yield better data, and publication/reproducibility concerns may require running the full 40 cycles to completion.
Tip
If adding extra cycles (more than 40) is desired, be sure that you run excellent negative controls since nonspecific amplification is a concern.
Unless the assay has severe issues, amplification graphs will be a sigmoidal curve. Shallow curves show poor amplification, with steep ones being optimal. Different dyes will give different results depending on factors such as template base pairs and stability. Corrupted sample material can be an issue as well.
Tip
If amplification efficiency is a major issue, this can be caused by degraded samples, those high in inhibitors, or excessively long amplicons. In those cases, droplet digital PCR (ddPCR) can give significantly greater precision and better results than qPCR.³
Optimization, Validation, and Challenges
Depending on the experimental goals and sample variability, an assay protocol will likely need to be adjusted during initial development and in response to non-ideal results.
Controls
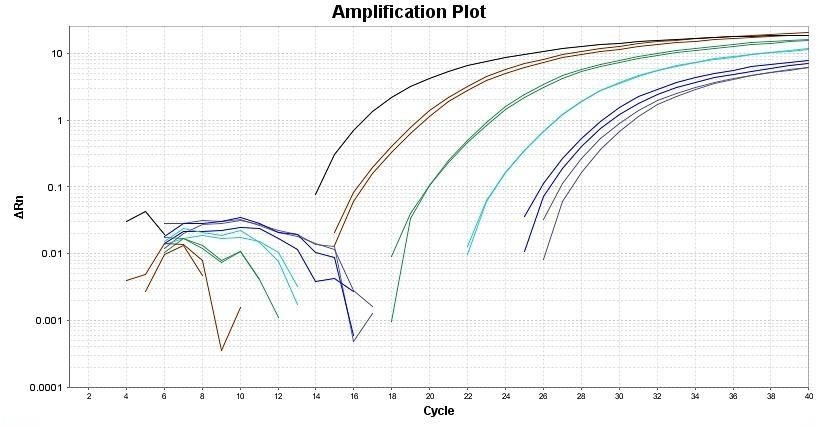
Including controls in a qPCR procedure is essential to experimental best practices, expected by MIQE guidelines and any reputable peer-reviewed journal dedicated to scientific reproducibility.
The four basic qPCR controls are:
- Extraction control- a sample that has been extracted before and has had positive results. Keep some sample matrices from prior successful extractions. This is a useful measure of whether or not the current target sample has been successfully extracted and to what extent.
- Positive control- includes a sample that is known to express well. This should always give a dependable signal, which assists in normalization and identifying any major issues with the overall procedure.
- Negative control-includes base reagents and water, but no sample. This should always have no signal, but it can often indicate sample contamination or poor experimental protocols if it does.
- No-template control (NTC)-includes everything (mix, etc.) except the template. This can also be useful for normalization but is most frequently used for identifying any inhibitors present or issues with the template itself.
Ideally, every type of control will be used in every experiment, but not every type will apply to each procedure. If a previously-validated assay is going to be run with a new primer or probe, revalidation is unlikely to be required, but run a positive control to confirm that it is working.
Differences in background fluorescence can lead to differences in fluorescence levels of individual wells. By subtracting this, we can increase the precision of our technical replicates. This is one of the main reasons passive reference dyes are so widely used since they are a simple method of removing this background.
Figure 5 of a comparison of two intercalating dyes, as a researcher might run during the materials selection process of developing a new qPCR assay. In this case, the Q4ever™ Green dye shows a significantly brighter signal than SYBR® Green and will be the likely choice for an experiment. Once the dye has been chosen, the amplification curve can be processed further to eliminate the baseline (which is no longer necessary) and background fluorescence so that a more useful and precise curve can be seen.
Figure 6 shows a processed amplification plot of ΔRn the vs. number of cycles using the integrated instrument software. Verification by manually calculating one or two randomly-selected data points is recommended for unusual experiments or simply for emphasis on reproducibility.
Determining Ideal Annealing Temperature
Run a few sample assays using slightly different annealing temperatures. The usual range is between 55-65 °C, read at half-degree increments. This temperature gradient can then be analyzed using a standard curve to determine the optimal annealing temperature (Ta).
Tip
If your instrument does not have automatic software processing, or if you wish to confirm the optimal Ta manually, assess the Cq values at each temperature run using the positive control sample and choose the highest temperature before the Cq value begins to rise exponentially.
Many instruments have settings to make this process easier, usually only requiring information about the primer sequence and buffer ion concentrations.
Post-Amplification
For dye-based qPCR, it is essential to perform a melt curve analysis after the procedure to evaluate the amplification specificity. If there are extra lane bands when running a post-assay gel, than running a melt curve analysis (always expected, per MIQE standards2) is absolutely essential. When assessing the melt profile, all unknowns should show a single melt peak, with the NTC showing no peak at all.
By analyzing the melt curve and assessing at what temperature the melt peak occurs, you can determine to what extent off-target products are being produced. For example, primer-dimers are short, and so the Tm will be low, often within the 60s to low 70s, so an unexpected peak in that temperature range strongly suggests the presence of those secondary structures.
Note
If there is a single melt curve but with ‘double top’s, then that represents two distinct but similar amplification products. This usually represents either a pseudo-gene or splice products. This may or may not be an issue, for example when different variants of a single gene are expected.
If multiple peaks are present, then redesign the assay with a new primer set, or raise the Ta slightly to see if the off-target product can be eliminated. Higher annealing temperatures improve specificity in many cases, although the assay will be slightly less efficient.
Statistics and Calculations
Once the qPCR procedure has been completed, the researchers’ work will take them away from the bench for the next phase: analyzing the gathered data to assess the success of the assay. In general, all calculations should be included in publications to the greatest extent permitted by length limitations.
For common types of analysis, many open-access calculators exist, and many other equations can simply be named without having to show every line of the work. What calculations are necessary, and the extent to which they need to be shown, will vary widely based on the assay and publication requirements.
Relative Gene Expression
To calculate gene expression, calculate the difference between Cq from the control vs. the treated sample. The ΔCq will then permit a comparison to show the relative difference.
ΔCq = Cq(treated) - Cq(control)
Relative Quantity (RQ) refers to a full change in expression. It is calculated very simply:
RQ = 2-ΔCq
However, to calculate well, we need to use a reference gene to show variances in sample volumes and quality. Reference genes are usually ‘housekeeping’ genes and will dependably express. This will permit the calculation of the Δ-ΔCq.
Δ-ΔCq is the difference of Cq (target minus treated) and Cq(reference genes). It only works when the assay amplifies the target at 100% efficiency. This is known as the Livak equation.4
Other equations include other variants, as well as normalization for multiple reference genes. For data that may not be normally distributed, when it is skewed to the either left or right, a Shapiro-Wilk normality test may be required. This determines the likelihood that the expression values do not represent a normal curve.
Common Types of Comparative Analyses
Students' T Test: Compares two groups, to determine of the averages of the two samples differ, and it assumes normal distribution of each. This is frequently seen in graphs that compare a control group vs. a treated group.
Analysis of Variance (ANOVA): compares more than two groups, and assumes normal distribution of means as well as equal variance. A common use is in showing individual comparisons between populations. It is a very popular method of analysis. The two types of ANOVA are the one-way and two-way. If the experiment is only testing one variable, no matter how many groups (such as in a time course), that is a one-way ANOVA. A two-way ANOVA will include more than one variable.
Data Analysis and Visualization
For qPCR data analysis, bar graphs, box-and-whisker, and dot plots are the most common methods of visualizing data sets.
- Standard deviation (SD) measures the dispersion of a dataset relative to its mean, and represents the variability of the data set. It does assume a normal distribution of data.
- The standard error of the mean (SEM) measures how much discrepancy there is likely to be in a samples' average compared to the population average. It is essentially the standard deviation of the two averages. It is intended to demonstrate the precision of the data, and will always be smaller than the SD. To calculate this fully, the experiment must be run multiple times and the standard deviation calculated each time. An easier method simply calculates the SEM by dividing the standard deviation by the square root of the number of samples.
- Bar graph: easily shows the mean/average, as well as the standard error. It does not provide any information on the relative distribution of the data, however.
- Box-and-whisker: shows median value, upper and lower quartiles, as well as min and max value, and outliers. However, it doesn't show anything about the average or the error.
- Dot Plot: shows individual data points are all shown, permitting full display of the results to the reader. It can be difficult to assess easily, however.
Multiplex and Multi-Plate Analysis
Multiplex assays nearly always require extra time and work to validate at the beginning. Reagents are often difficult to choose, and often must be added in higher concentrations. When running any multiplex procedure, make all possible efforts to minimize any negative interactions. Run the assays in various combinations and assess if the Cq values are similar, which represents low to no interference. If those values vary widely, then those cannot be run in the multiplex assay. Additionally, be sure to assess the spectral overlap of the selected fluorescent dyes carefully.
Variability between plates may be caused by user error, sample degradation, differences in measured concentration, instrument fluctuation, or even degradation of PCR components due to improper or extended storage.
The number of samples and targets must be considered, and should include replicates as necessary as well as controls. If only using a single plate (if all will fit into a single plate) then no inter-run calibration will be necessary.
Tip
Technical replicates increase precision by identifying outliers. The usual standard deviation value for replicates is between 0.2-0.3.
If using two or more plates, if there are direct comparisons, no inter-run calibrations are required, since all plate-to-plate differences will be identical.
However, if the plates encompass no direct comparisons, then there must be an inter-run calibration. This is often necessary with larger experiments, where it is impossible to have references on every plate, or where samples must be divided between plates. This is not an issue, as long as an inter-run calibrator is run on every plate to allow for variability.
There are several types of inter-run calibrators. Pooled nucleic acid samples are common, or reference materials such as stock cDNA or linearized plasmids.
Frequent Challenges
One of the most frequent preventable errors made, leading to multiple retracted papers, is inaccurate Cq calculation. Cq and Ct values are technically different, but are frequently used interchangeably. Cq stands for 'quantification cycle'. A Ct value is a 'threshold cycle'. There is Top (take-off point) as well, but Cq is the most common and best-accepted.
It is almost always possible to calculate the Cq values automatically with the instrument, but in the interest of reproducibility and for publication standards of extremely strict journals, doing the calculations manually as well may be required for verification.
Tip
A good rule of thumb is to set the Cq point to no more than 10 times the SD of the threshold noise.
If your instrument has the Cq calculations showing an unexpected value (far lower or higher than estimated), there is likely a deeper issue. Confirm that the positive and negative controls are performing as expected.
Another common issue is not taking the template sample type into account. A well-performing protocol for one type of sample may or may not behave equally well for another kind. Adjustments or substitutions are likely to be necessary, even if the original protocol can serve as an excellent starting guideline.
Example amplification curves for the same test run using different kinds of DNA
As is clear from the above two amplification plots, although the observed data is similar to an extent, the type of DNA has a major effect on the procedure. Always be sure to confirm if the selected materials are compatible with the sample type you will be using, such as genomic, plasmid, viral or cDNA templates.
If assay signal is low, one possible cause is suboptimal primer concentration.
Tip
Try going outside the usual concentrations of 100-500nM.
It may be necessary to run some test amplifications to find a balance between low concentrations (and commensurate low signal) and the production of off-target products (such as primer-dimers) at high concentrations.
Summary and Closing Remarks
The qPCR assay is a core procedure with ever-expanding applications for researchers. It has contributed to significant advances in disease detection, gene expression, genotyping, and cloning, among many other applications.
If researchers want to multiplex multiple targets per well or have multiple similar targets, probes might be the best choice. However, if cost is an issue, then intercalating dyes might be a more economical choice.
The procedure itself is highly adaptable but requires care and consistency to ensure dependable and reproducible results. By ensuring that all necessary optimization steps and controls are represented, a researcher can be confident in the validity of their amplification.
References
- A Basic Guide to Real Time PCR in Microbial Diagnostics: Definitions, Parameters, and Everything. Petr Kralik and Matteo Ricchi. Front. Microbiol., 02 February 2017 | https://doi.org/10.3389/fmicb.2017.00108
- Stephen A Bustin, Vladimir Benes, Jeremy A Garson, Jan Hellemans, Jim Huggett, Mikael Kubista, Reinhold Mueller, Tania Nolan, Michael W Pfaffl, Gregory L Shipley, Jo Vandesompele, Carl T Wittwer, The MIQE Guidelines: Minimum Information for Publication of Quantitative Real-Time PCR Experiments, Clinical Chemistry, Volume 55, Issue 4, 1 April 2009, Pages 611–622, https://doi.org/10.1373/clinchem.2008.112797
- Taylor, S.C., Laperriere, G. & Germain, H. Droplet Digital PCR versus qPCR for gene expression analysis with low abundant targets: from variable nonsense to publication quality data. Sci Rep 7, 2409 (2017). https://doi.org/10.1038/s41598-017-02217-x
- Livak KJ, Schmittgen TD. Analysis of relative gene expression data using real-time quantitative PCR and the 2(-Delta Delta C(T)) Method. Methods. 2001 Dec;25(4):402-8. doi: 10.1006/meth.2001.1262. PMID: 11846609.