Cell Lysis, Dialysis & Desalting Reagents
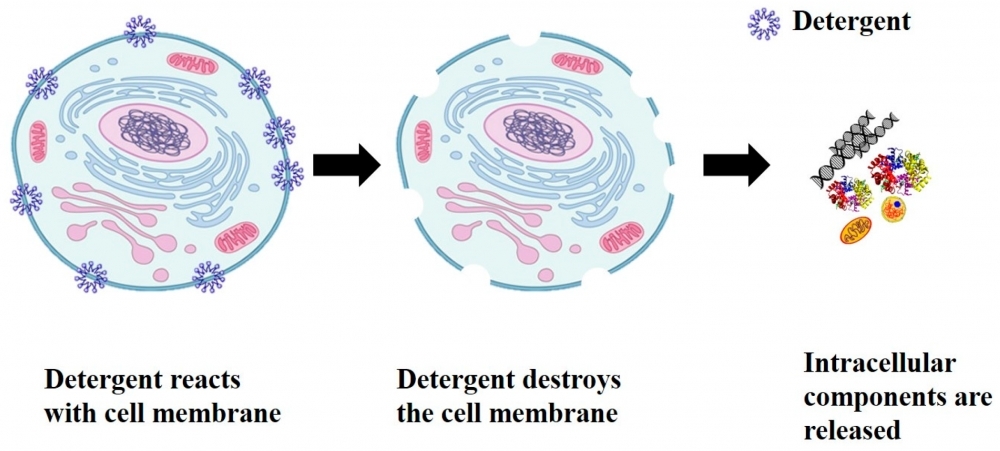
Cell lysis refers to the breaking down of cells, and it is often used to analyze specific cellular compositions such as proteins, lipids, nucleic acids, reporter molecules, cell signal molecules and other small biomolecules. Depending upon the detergents used, either all or some membranes are lysed.
Lysis Reagents
Chemical structure for ReadiUse™ 10% Triton X-100 *Hydrogen Peroxide-and Carbonyl-Free*. Triton X-100 is one of the most common cell lysis detergents.
For example, NP-40 is common for extraction of proteins from muscle tissues and mammalian cell lines since it is a weaker detergent that can solubilize proteins but not denature them. Alternatively, RIPA lysis buffer may be preferred depending on experimental need since it can release most protein types from cultured cells while also disrupting many weak noncovalent protein-protein interactions. Though a handful of lysis buffers have been reported for use across several different proteomic techniques, it has proven difficult to design a lysis buffer that can effectively isolate a broad assortment of proteins.
Table 1. Common Mammalian Cell Lysis Buffers for General Protein Extraction
Buffer ▲ ▼ | RIPA Lysis Buffer ▲ ▼ | NP-40 Lysis Buffer ▲ ▼ |
Purpose | Recommended for the extraction of cytoplasmic, nuclear, membrane-bound and mitochondrial proteins. It contains harsh denaturing, ionic detergents and milder non-ionic detergets. Disrupts protein-protein interactions. | Recommended for extraction of cytoplasmic and membrane-bound proteins. It is a milder, non-ionic detergent. Proteins retain their native state, and protein-protein interactions are preserved. |
Composition |
|
|
Compatible protein assays | BCA assays | BCA assays |
Recommended applications | SDS-PAGE, Western blot | ELISA, protein electrophoresis, Western blot |
There are a handful of criteria that make each lysis buffer successful in an experiment, the first being the presence of detergents or denaturants. Many cell lysis buffers contain a detergent that must be able to simultaneously disrupt the lipid bilayer of the cell membrane while preserving the integrity of the target molecule. Such detergents can disintegrate both hydrophobic and hydrophilic molecules in the cell membrane and disrupt protein-protein or protein-lipid interactions within the cell. For these reasons, detergents are available in varying strengths, and are normally incorporated into a procedure or chosen based on whether extracted proteins need to be denatured or not.
Based on their charge carrying capacity, detergents are generally divided into three groups: cationic, anionic and non-ionic. Though detergents are most widely used for lysing mammalian cells, they can also be used with lysozymes for lysing bacteria if, of course, there is a step in the procedure where the bacterial cell wall is broken down first. For mammalian cells, non-ionic detergents are commonly preferred as they tend to cause less damage to proteins and enzymes. Many types of non-ionic detergents exist, and some of the most popular include CHAPS and CHAPSO, both zwitterionic, along with Triton-X and those in the Tween series. Some ionic detergents, like sodium dodecyl sulfate (SDS), are also widely used for lysing cells because of the inherent high affinity to bind and denature proteins quickly. Ionic detergents are commonly used if downstream gel electrophoresis methods are required. Similarly, chaotropic agents can also be used for cell lysis. Some examples include urea and guanidine-based substrates which can destroy the hydrogen bond of water in solutions, thereby weakening hydrophobic interactions. This, in turn, influences the stability of other macromolecules in the solution, like nucleic acids and proteins, by weakening their hydrophobic effect.
Table 2. Properties and main applications of common detergents.
Detergent ▲ ▼ | Properties ▲ ▼ | Type ▲ ▼ | Aggregation No. ▲ ▼ | MW mono (micelle) ▲ ▼ | CMC mM (%w/v) ▲ ▼ | Cloud point °C ▲ ▼ | Dialyzable ▲ ▼ | Application ▲ ▼ |
SDS (sodium dodecyl sulfate) | Denaturing, strong lysis agent | Anionic | 62 | 18,000 | 6-8 (0.17-0.23) | >100 | No | Cell lysis, Electrophoresis, WB, Hybridization |
Triton X-100 | Non-denaturing, mild lysis agent | Non-ionic | 140 | 90,000 | 0.24 (0.0155) | 64 | No | Cell culture, Enzyme immunoassays, IP, Membrane protein solubilization |
Triton X-114 | Non-denaturing, mild lysis agent | Non-ionic | - | - | 0.21 (0.0113) | 23 | No | Cell lysis, Various routine protein and molecular biology techniques |
NP-40 | Non-denaturing, mild lysis agent | Non-ionic | 149 | 90,000 | 0.29 (0.0179) | 80 | No | Isoelectric focusing |
n-dodecyl-Β-D-maltoside | - | Non-ionic | 98 | 50,000 | 0.17 (0.009) | - | No | Protein Crystallization |
Brij 35 | - | Non-ionic | 40 | 49,000 | 0.09 (0.011) | >100 | No | Cell lysis, Chromatography, Electrophoresis, HPLC, Membrane protein solubilization |
Digitonin | Non-denaturing, mild lysis agent | Non-ionic | 60 | 70,000 | - | - | No | Membrane solubilization, Protein solubilization, Enzymology, Chromatography, Cell culture |
Tween-20 | Mild lysis agent | Non-ionic | - | - | 0.06 (0.0074) | 95 | No | Cell lysis (mammalian cells), WB, ELISA, Enzyme immunoassays, Membrane protein solubilization |
Tween-80 | Mild lysis agent | Non-ionic | 60 | 76,000 | 0.01 (0.0016) | - | No | ELISA, WB, Immunoassays |
Octyl-beta-Glucoside | Non-denaturing, mild lysis agent | Non-ionic | 27 | 8,000 | 23-24 (∼0.70) | >100 | Yes | Membrane protein solubilization |
Additionally, pH is also a major factor in an efficient lysis buffer as the pH heavily influences the physical state of the proteins within the sample. Inherently, different proteins have different ranges of pH in which they are stable or will actively bind to, or aggregate with, other proteins. In an ideal lysis buffer, the pH of the solution should be within 1.0 pH unit of the protein's isoelectric point (pI). At the pI, the protein has no net charge, which means that if the pH of the buffer equals the pI of the protein, the protein will be neutral and will not adversely aggregate or precipitate. Ionic strength, too, is a key part of any successful lysis buffer.
Salts aid lysis by locally changing the pH of cells, thus facilitating denaturation of cellular components. Salts, like sodium and potassium chloride, (NaCl or KCl) can increase the ionic strength of the buffer. Similarly, metal ions, ligands, and glycerol can be added to the lysis buffer to increase protein solubility and stability. Additionally, metal chelators such as EDTA and EGTA can be included to reduce oxidation damage and chelate metal ions, which may impact the ionic strength.
Lastly protease inhibitors can be included into the lysis buffer to improve protein stability and/or prevent proteolysis of a sample. Protease inhibitors are chemical or biological compounds that can reversibly or irreversibly inhibit the action of proteases, which can break down the target protein. For protease inhibitors, size and molecular weight vary widely, and they can exist as small chemicals, peptides, or even other proteins. Because of the diversity, protease inhibitors are often grouped by the type of protease they inhibit and serine, threonine, aspartic acid, and cysteine inhibitors are common.
Tools: |
Lastly protease inhibitors can be included into the lysis buffer to improve protein stability and/or prevent proteolysis of a sample. Protease inhibitors are chemical or biological compounds that can reversibly or irreversibly inhibit the action of proteases, which can break down the target protein. For protease inhibitors, size and molecular weight vary widely, and they can exist as small chemicals, peptides, or even other proteins. Because of the diversity, protease inhibitors are often grouped by the type of protease they inhibit and serine, threonine, aspartic acid, and cysteine inhibitors are common.
Table 3. Properties of common protease and phosphatase inhibitors.
Inhibitor ▲ ▼ | MW (kDa) ▲ ▼ | Protease/Phosphatase Inhibited ▲ ▼ | Type ▲ ▼ | Solubility (solvent) ▲ ▼ | Final Conc. in Lysis Buffer ▲ ▼ | Stock (store at -20°C) ▲ ▼ |
Aprotinin | 6511.5 | Trypsin, chymotrypsin, plasmin | Reversible | 10 mg/mL (H2O) | 2 µg/mL | Dilute in water, 10 mg/mL. Do not re-use thawed aliquots. |
Leupeptin | 475.6 | Lysosomal | Reversible | 1 mg/mL (H2O) | 5-10 µg/mL | Dilute in water. Do not re-use thawed aliquots. |
Pepstatin A | 685.9 | Aspartic proteases | Reversible | 1 mg/mL (MeOH) | 1 µg/mL | Dilute in methanol, 1 mM |
PMSF | 174.2 | Serine, cysteine proteases | Reversible | 18 mg/mL (MeOH) | 1 mM | Dilute in ethanol. You can re-use the same aliquot. |
EDTA | 372.2 | Metalloproteases that require Mg2+ and Mn2+ | Reversible | 10 g/100 mL (H2O) | 5 mM | Dilute in dH20, 0.5 M. Adjust pH to 8.0. |
EGTA | - | Metalloproteases that require Ca2+ | - | - | 1 mM | Dilute in dH20, 0.5 M. Adjust pH to 8.0 |
Sodium fluoride | 42.0 | Serine/threonine phosphatases | Irreversible | 40 mg/mL (H2O) | 5-10 mM | Dilute in water. Do not re-use once defrosted. |
Sodium orthovanadate | 183.9 | Tyrosine phosphatases | Irreversible | 20 mg/mL (H2O) | 1 mM | Dilute in water. Do not re-use once defrosted. |
Dialysis Reagents
Simplified illustration of basic dialysis principle, showing the beginning and endpoint stages of the technique. The dialysis membrane containing the initial concentrated solution permits controlled diffusion into the surrounding solvent, eventually resulting in equilibrium. Figure made in Biorender.
Dialysis is a common laboratory technique used to remove contaminants in a solution by selective and passive diffusion through a semipermeable membrane. Dialysis is defined as the diffusion based, size selective, transportation of molecules from a higher concentration to lower one, the end goal being equilibrium. Dialysis is commonly used to remove small, contaminating molecules like salts, reducing agents (e.g. dithiothreitol [DTT] and 2-mercaptoethanol [BME]), preservatives (e.g. sodium azide and thimerosal), and non-reacted crosslinking or labeling reagents (e.g. sulfo-SMCC and biotin) from target proteins within a sample. Dialysis is a gentle but timely method and ultimately the selectivity and efficiency of dialysis is based on the chosen buffer and pore size of the semipermeable membrane.
The dialysis buffer is a reagent that comes in contact with the sample and must be chosen based on compatibility with other steps in sample preparation. Sometimes, the dialysate buffer can be used for stabilization of pH or redox potential to protect sensitive samples. Frequently, dialysis buffers include phosphate buffer, TRIS, MOPS, and HEPES, though in the simplest case water, too, can be utilized. Other components can be added to the dialysis buffer to increase the activity of macro-micro molecular separation, including amino acids, EDTA, inhibitors, activators, cofactors, or DTT. The dialysis membrane also plays a key role in active dialysis, which relies on the ratio of membrane area to sample volume, pore size, and porosity.
Table 4. Comparison of nitrocellulose and polyvinylidene fluoride membranes.
▲ ▼ | Nitrocellulose (NC) ▲ ▼ | Polyvinylidene Fluoride (PVDF) ▲ ▼ |
Protein binding capacity | 80 to 100 µg/cm² | 170 to 200 µg/cm² |
Binding interactions | Proteins bind to membrane through hydrophobic interactions | Proteins bind to membrane through hydrophobic and dipole interactions |
Physical characteristics | Brittle, fragile and not chemically resistant | Physically durable and chemically resistant |
Pore size | 0.1, 0.2 or 0.45µm | 0.1, 0.2 or 0.45µm |
Membrane format | Pre-wetted with methanol | No need to pre-wet |
Attributes |
|
|
Generally, a larger area to sample volume ratio, higher porosity, and larger pore size increases the speed of dialysis. The selected pore size of the membrane should be as wide as possible while still retaining the desired sample substance; in general, the molecular weight cutoff (MWCO) should be about twice the size of the dialyzed sample. Importantly, membranes may have the ability to bind substances in a nonspecific manner, potentially causing loss of the desired sample. This can especially happen in samples that contain proteins with very different binding affinities. For this reason, regenerated cellulose and cellulose esters are commonly used materials for membranes as they exhibit low binding for many substances, specifically proteins. Other common synthetic dialysis membrane materials include polysulfone, polyethylene, polypropylene and polyvinylidene fluoride.
Datasets: | Tools: |
Desalting Reagents
Desalting is a process that refers to the removal of salts from a sample, and is commonly combined with buffer exchange, the replacement of one set of buffer salts with another. Desalting is a fast and simple technique used to purify biomolecules from salts and small molecules, and most protein purification workflows require desalting, buffer exchange, or both. Like dialysis, desalting can remove other low molecular weight molecules like cofactors, inhibitors, contaminants, labeling/derivatization reagents, unreacted radioactive labels, phenol, free nucleotides, primers, or dyes from a protein sample. Desalting is necessary since such small molecules may interfere with peptide ionization and add to the chemical noise or background in downstream analysis.
Many desalting kits are commercially available that often come with their own buffer which is highly efficient in removing many different salts including, but not limited to, MgCl2, NaCl, KCl, CaCl2, LiCl and CsCl. For samples carrying charged groups, an eluent containing a buffer salt may also be included. Usually, NaCl is often a go-to component in desalting buffers, and such normally have salt concentration of at least 150 mM to prevent possible ionic interactions with the medium. Buffers with salt concentrations over 1 M have also been reported for use in certain applications, though at this molarity hydrophobic substances can be retarded through the column or unintentionally bind to the resin bed. Regardless, the desalting buffer should be compatible with both the target molecule and the desalting column matrix. As with dialysis and lysis buffers, it is critical that the pH be maintained within optimal range for the target molecule's stability.
Desalting columns are another important factor that determines the efficacy of the procedure. Desalting columns are a kind of gel filtration chromatography column that contains a resin bed that captures specific components of the sample. Like dialysis membranes, the maximum effective pore size (MWCO) of the resin within the column determines which molecules separate from the sample. Molecules that are significantly smaller than the MWCO penetrate the pores of the resin and are then excreted, while molecules larger than the MWCO are unable to enter the pores and remain stuck in the volume of the column. Some traditional resin materials include dextran, cellulose, polyacrylamide, and polyacrylamides. Notably, synthetic Sephadex resins have solidified their place as the mainstay material for desalting columns due to resilience and tunable pore size. Though loose resins are available, prepacked formats save time, increase ease of use and reproducibility in results. Additionally, there are many types of desalting columns on the market, including those designed for simple gravity flow, spin columns designed for centrifugation, or pressure-compatible columns designed for pump applications.
Table 5. ReadiUse™ Desalting Columns
Cat# ▲ ▼ | Product Name ▲ ▼ | Unit Size ▲ ▼ |
60504 | ReadiUse™ Disposable PD-10 Desalting Column | 5 Columns |
60505 | ReadiUse™ Disposable PD-10 Desalting Column | 10 Columns |
Product Ordering Information
Table 6. ReadiUse™ Lysis Buffers
Cat# ▲ ▼ | Product Name ▲ ▼ | Unit Size ▲ ▼ |
20012 | ReadiUse™ mammalian cell lysis buffer *5X* | 10 mL |
24100 | ReadiUse™ bacterial cell lysis buffer *5X* | 10 mL |
24102 | ReadiUse™ Yeast Cell Lysis Buffer *5X* | 10 mL |
37012 | ReadiUse™ RBC Lysis/Fixation Solution | 50 mL |
37013 | ReadiUse™ RBC Lysis/Fixation Solution | 100 mL |
37014 | ReadiUse™ RBC Lysis Buffer | 50 mL |
60015 | ReadiUse™ Viral RNA Lysis Buffer | 50 mL |
60016 | ReadiUse™ Viral RNA Lysis Buffer | 500 mL |
References
Solubilization of Proteins: The Importance of Lysis Buffer Choice
Protease Inhibitors 101: How They Work and How to Use Them
A Review on Macroscale and Microscale Cell Lysis Methods
Sample dialysiss in the lab
A comparative study on methods of optimal sample preparation for the analysis of oligonucleotides by matrix-assisted laser desorption/ionization mass spectrometry
Strategies In Protein Purification