Cell/Cytoplasmic Membrane Potential Activity & Analysis
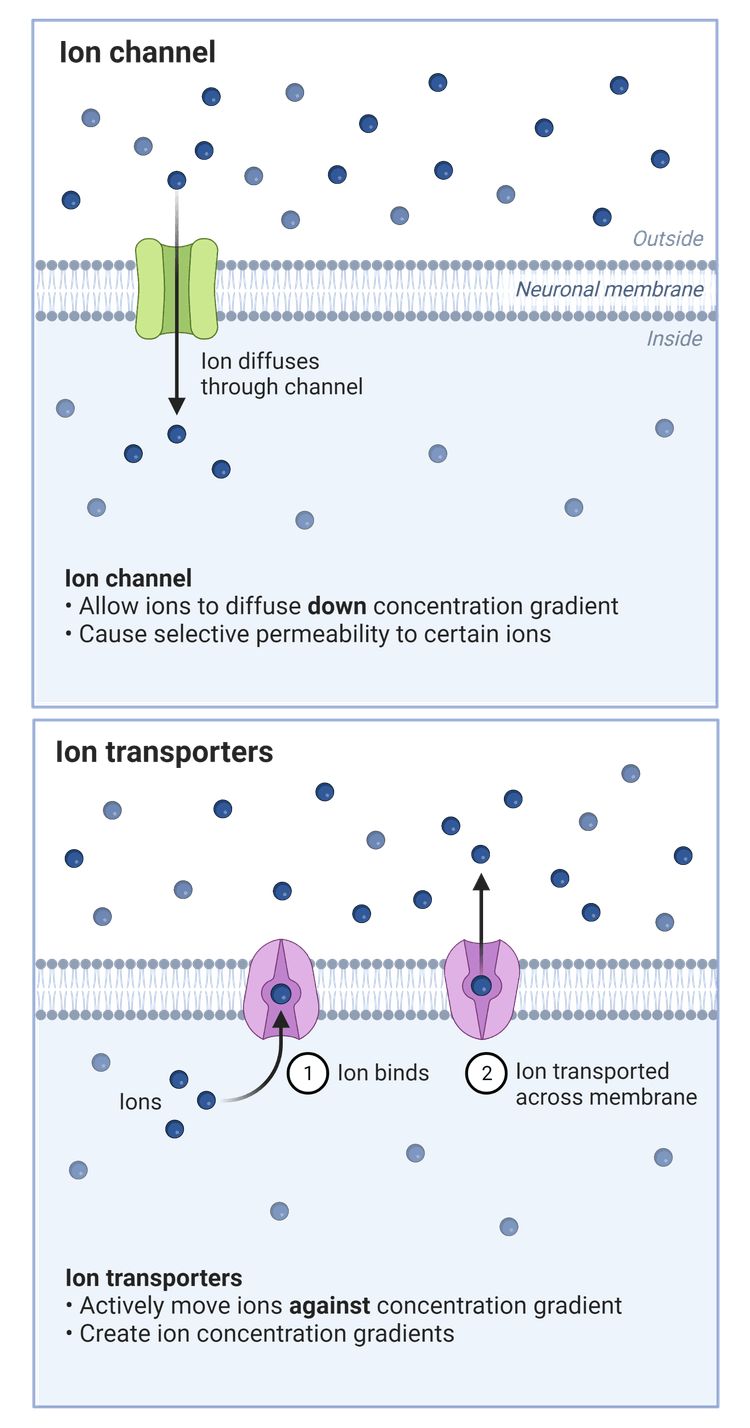
Ions flow across the cell membrane depending on their charge; positive ions are attracted to the negative side of the membrane and vice versa. Generally, the intracellular space of cells and organelles are negative in relation to the external medium. This means electrical forces will guide the flow of positively charged ions (cations) like sodium (Na+), potassium (K+), and calcium (Ca2+) into the cell, and escort negatively charged ions (anions) like chloride (Cl-) out. In the case that there is no net ion current, the excitable membrane is considered stable.
Only live cells can maintain MP, making MP one of the most commonly used parameters to determine cell viability as it represents the differential distribution of ions across a biological membrane.
- Hyperpolarization is the shift in a cell's MP to a more negative value, which correlates to an increase in transmembrane potential that generally insinuates an increase in cell activity.
- Depolarization is the opposite effect, correlating to a decrease in transmembrane potential and cell activity, although it does not always imply cell death.
Significance of Membrane Potential in Various Cell Types
Bacterial Cells
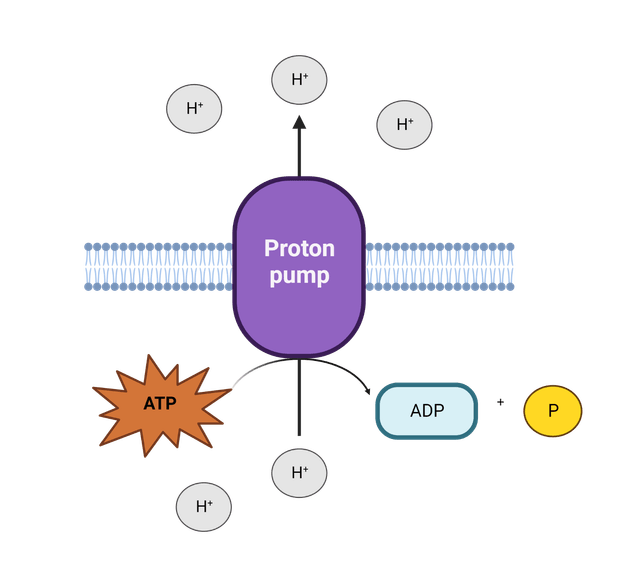
Cell division requires MP for identifying the correct location of the division site, which may be inhibited by native protonophores. Due to the central, dynamic, role MP plays in bacteria, the bacterial cytoplasmic membrane is a major target for antimicrobial compounds like membrane-targeting peptides. Disrupting membrane function, can therefore, simultaneously inhibit one, or several of these essential processes.
Neurons
Throughout the brain, neurons readily react to the production of electrical impulses, a downstream result of cellular MP. The cell's interior has a resting MP of roughly -60mV, and maintaining this steady state is critical for upholding the cell's physiology. When the resting MP decreases to a more negative state, a voltage-gated cation channel will open. This cationic channel is established by ATP-dependent pumps including those composed of sodium-potassium antiporters.
As the cations move across the plasma membrane the MP changes which influences the generation of an action potential. The resulting action potential propagates throughout the cell to the axon, and when it finally reaches the axonal terminal, it will cause depolarization of neighboring cells through synapses. As a whole, the propagation of impulses caused by MP upstream is what allows synaptic communication along nerve fibers. Disruption of this mechanism may have drastic effects that result in the lack of impulse generation and conduction, which are easily illustrated by neurotoxins and demyelinating disorders. Aside from sodium potassium pumps, calcium channels are also essential to the brain, and function as neurotransmitter release controls and to mediate depolarization-induced repetitive spikes, among other things. Voltage gated calcium channels are also involved in regulating cell excitability, gene transcription, and synaptic transmission.
Skeletal Muscle
The stimulus for skeletal muscle activity is always derived from nerve impulses, which mean muscle fibers are also electrically excitable by MP. The muscle fiber cell membrane, known as the sarcolemma, consists of ion channels and pumps that can maintain a resting MP in the range of -70 to -90 mV. The resting MP of a skeletal muscle cell receives a significant influence from chloride ion conductance as these ions play a big role in physiology, and help to maintain muscle activity during repeated stimulation.
Upon muscle contraction, potassium ions leak from the cell. With repeated activity, potassium ions will run down the concentration gradient across the sarcolemma. If chloride ions were not present to maintain the resting MP, muscle tissue could not repolarize sufficiently. This would prevent channels from regenerating into an active state, which is necessary for succeeding action potentials that impact and trigger myofilament interaction. Calcium channels are significant in the muscular system, as well, as they function to allow smooth muscle cell contraction, a critical feature of the cardiac conduction system. As calcium helps to coordinate the contractile activity of muscle cells, it aids in controlling the supply of ATP when needed, specifically for glycogenolysis and the glycolytic pathway. Skeletal muscle constitutes around 40% of muscle mass, so dysregulation of muscle function results in profound systemic and physiological disorders, including neuromuscular jitters and amyotrophic lateral sclerosis (ALS).
Table 1. Screen Quest™ Calcium Assays for screening GPCR and calcium channel targets.
Calcium Assay ▲ ▼ | Ex (nm) ▲ ▼ | Em (nm) ▲ ▼ | Cutoff (nm) ▲ ▼ | Unit Size ▲ ▼ | Cat No. ▲ ▼ |
Screen Quest™ Fluo-4 No Wash Calcium Assay Kit | 490 | 525 | 515 | 10 plates | 36325 |
Screen Quest™ Fluo-4 No Wash Calcium Assay Kit | 490 | 525 | 515 | 100 plates | 36326 |
Screen Quest™ Fluo-8 No Wash Calcium Assay Kit | 490 | 525 | 510 | 1 plates | 36314 |
Screen Quest™ Fluo-8 No Wash Calcium Assay Kit | 490 | 525 | 510 | 10 plates | 36315 |
Screen Quest™ Fluo-8 No Wash Calcium Assay Kit | 490 | 525 | 510 | 100 plates | 36316 |
Screen Quest™ Fluo-8 No Wash Calcium Assay Kit | 490 | 525 | 510 | 100 plates | 36316 |
Screen Quest™ Fluo-8 Medium Removal Calcium Assay Kit *Optimized for Difficult Cell Lines* | 490 | 525 | 510 | 1 plates | 36307 |
Screen Quest™ Fluo-8 Medium Removal Calcium Assay Kit *Optimized for Difficult Cell Lines* | 490 | 525 | 510 | 10 plates | 36308 |
Screen Quest™ Fluo-8 Medium Removal Calcium Assay Kit *Optimized for Difficult Cell Lines* | 490 | 525 | 510 | 100 plates | 36309 |
Screen Quest™ Calbryte-520 Probenecid-Free and Wash-Free Calcium Assay Kit | 490 | 525 | 515 | 1 plates | 36317 |
Common Methods of Membrane Potential Analysis
Fluorescent and Ratiometric Dyes
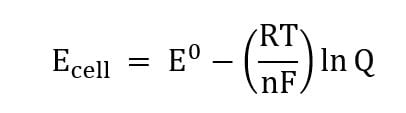
Fluorescent microscopy can be performed at different stages of the experiment, specifically before and after the introduction of the dye. The electric potential difference across the plasma membrane can be calculated (before and after dye addition) using gas constant, temperature, valence of the charged molecule, Faraday's constant, with external and internal concentrations of the charged molecule. The Nernstian dye method does possess some drawbacks as permeabilities tend to be lower with gram-negative bacteria, and these studies may require a treatment with EDTA to chelate divalent cations. Low permeability also means this method is not suitable for capturing fast dynamics. When used at high concentrations, Nernstian dyes can also become invasive to cellular MP.
ANEP dyes are another common fluorescent dye that respond to electrical potential changes. They are ultra fast-responding, and can provide millisecond response time. ANEP dyes function by responding to electric changes in their environment. These dyes can essentially shift their excitation spectra according to MP in response to electrical changes in the microenvironment. When the surrounding electric field shifts, ANEP dyes will alter their electronic structure and exhibit consequently fluorescent properties. In addition to other studies, ANEP dyes have been documented for use in MP activity analysis for single neurons, cardiac cells, intact brains, and in studying bacteria. This dye method, however, suffers from a number of drawbacks, including a weak signal-to-noise ratio. Additionally, the magnitude of their potential-dependent fluorescence changes frequently, as this measurement is dependent upon MP, which can make analysis and true quantification tricky.
Flow Cytometry
Flow cytometry has become more readily used to estimate MP in eukaryotic cells in vitro, mitochondria in vitro and in situ, and in bacteria. Flow dyes are normally non-cytotoxic, do not block ion channels, and are not extruded by the glycoprotein efflux pump. A general protocol for use is simple: cells, probes, and reagents are treated, mixed, and incubated accordingly. Cells can then be visualized and quantified by using a flow cytometer, and statistical software can be used for analysis. Flow offers many advantages as testing is simple and relatively inexpensive, result times are rapid, and subpopulation of cells or an entire sample of cells as a whole can be quantified in parallel.
Patch Clamp
Patch-clamp electrophysiology can evaluate single ion channel conductance. In this method, first a microelectrode is sealed to a cell membrane and a patch of the cell membrane containing the ion channel of interest is either disrupted or removed. After a specified voltage is applied, the voltage forms a clamp, and membrane current, rather MP, may be measured. Patch-clamp methods are generally a less-frequently used technique in research, as they are highly invasive to cells, and can only measure one cell at a time.
Application Notes: | FAQs: |
Product Ordering Information
Table 3. Membrane Potential Assay Kits
Cat# ▲ ▼ | Product Name ▲ ▼ | Unit Size ▲ ▼ | Ex ▲ ▼ | Em ▲ ▼ |
35999 | Screen Quest™ Membrane Potential Assay Kit *Orange Fluorescence* | 1 plate | 535 | 560 |
36000 | Screen Quest™ Membrane Potential Assay Kit *Orange Fluorescence* | 10 plates | 535 | 560 |
36001 | Screen Quest™ Membrane Potential Assay Kit *Orange Fluorescence* | 100 plates | 535 | 560 |
36004 | Screen Quest™ Membrane Potential Assay Kit *Red Fluorescence* | 1 plate | 635 | 660 |
36005 | Screen Quest™ Membrane Potential Assay Kit *Red Fluorescence* | 10 plates | 635 | 660 |
36006 | Screen Quest™ Membrane Potential Assay Kit *Red Fluorescence* | 100 plates | 635 | 660 |
Table 4. Ordering Info for Slow Response Membrane Potential Probes Products
Table 5. Ordering Info for Fast Response Membrane Potential Probes Products