Investigating Intercellular Channels: Focus on Gap Junctions
Abstract
One of the main three types of intercellular junctions, gap junctions are crucial for several cell communication pathways, and any dysregulation can have immediate and lethal effects. They have been implicated in tumor development and the inflammatory response in general, as well as standard cellular regulation. Given this importance, being able to investigate gap junction activity is useful for several fields of study. Using fluorescent labels is one of the simpler ways to experimentally determine gap junction activity within a cell population. Fluorescent labels can be adapted and optimized for various cell lines, experimental setups, and instrument platforms. Either a flow cytometer or fluorescence microscope can be used to analyze gap junction activity.
Overview of Intracellular Channels
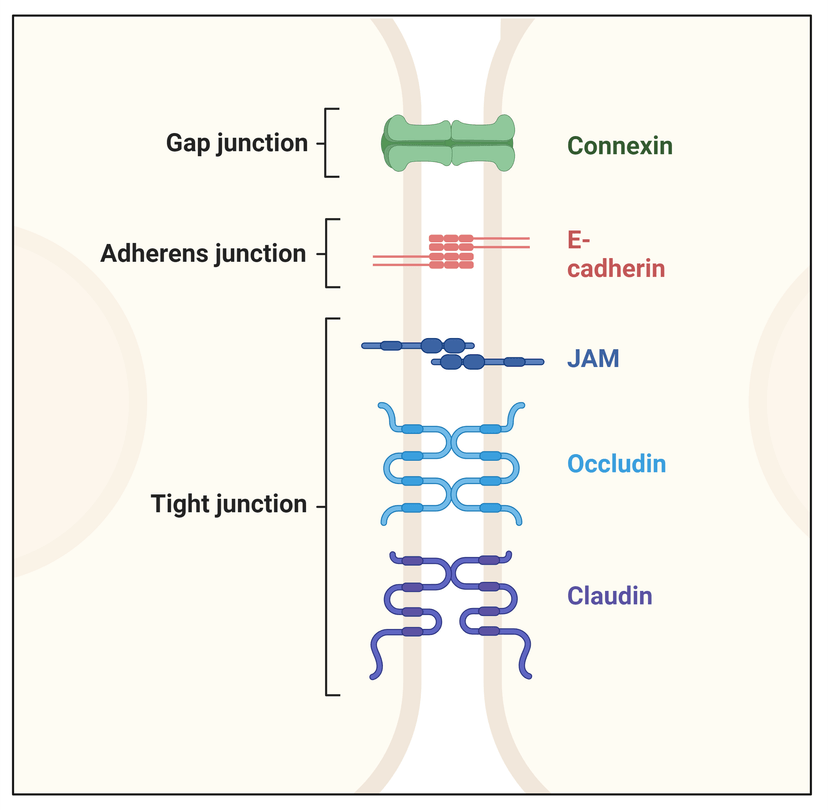
Common types of intercellular junctions, along with their primary composition material. Figure made in BioRender.
Tight junctions act as gates between cell surfaces, and are extremely dependent on cell polarity. Depending on subtype, they can be composed of varying proteins, such as claudin or occludin. Anchored within and through the plasma membrane, tight junctions do not directly transfer material between cells, but they are essential for protein signaling and intercellular crosstalk via protein-protein interactions. They also help regulate ion and other small molecular transfer between cells that provide a basis for cellular signaling. These signals control not only standard cell behavior such as migration, but also lifecycle actions like cell proliferation, differentiation, and death.
Adherens junctions are similar to tight junctions, as they are both anchored within the plasma membrane and transmit signals but not materials between cells. Adherens junctions are made primarily of E-cadherin transmembrane protein, and provide not only adhesive contacts for epithelial cells, but can bind to intracellular catenins. This facilitates the transfer of signals to organize the cytoskeletal structure as well as intracellular regulation of activities as important as gene transcription.
Gap junctions in particular are unique in that they are made of two interdocked cylinders made of connexin. Six of these molecules individually make up what is known as a hemichannel, which span the plasma membrane of each cell, facilitating easy transfer of small molecules when they meet and interlock to form a complete intercellular channel.
Unique Functionalities of Gap Junctions
Gap junctions are specialized intercellular membrane channels constituted with connexin that selectively facilitate the passage of small molecules of <1.2 kD across cells. They are tightly regulated by voltage, growth factors, cAMP, and retinoids, and they are modulated by phosphorylation. During cellular membrane depolarization (>+20mV) the channels will be open, but closed otherwise. Gap junctions have been implicated in inflammatory diseases and tumor behavior, and severe dysfunction results in cell death and multiple problems with the organism as a whole.
Since gap junctions transfer so many important molecular types (including second messengers as well as more typical yet essential ions) they are central in multiple regulatory cellular communication pathways that regulate cellular and tissue homeostasis. This importance is broadly termed gap junctional intercellular communication (GJIC) and has implications up to and including organ health as well as cell behavior and maintenance.
Principles of Gap Junction Analysis via Fluorescent Labeling
Simplicity is the key to experimental setup. The cell population is divided evenly, with one population incubated with a low-toxicity cytoplasmic dye. This dye will be contained within those living cells. The other population will be stained with a plasma membrane dye in an alternative color. A wide spectral separation between the colors is ideal, to minimize signal overlap. After washing and mixing, any of a variety of experimental stimuli can be applied (or simply a period of time will be permitted to pass) and the mixed population of cells can be either analyzed via flow cytometry or simply visualized with a fluorescence microscope.
The possible results can be grouped into four possibilities: double-positive cells showing both colors of dye are those that have exchanged cytoplasmic material via gap junction. Double-negative cells (no staining at all) are dead, and are a useful control group as well as a measure of the overall health of the population. Other populations will be stained only with the colors of the dyes they were incubated with, and these single-signal cells are then used to compare to the double-positive cells, with that proportion being a determination of gap junction activity.
Exemplifying this type of noninvasive gap junction investigation, the Cell Meter™ Fluorescence Gap Junction Tracing Kit provides a reliable and robust assay for the in vitro determination of gap junction function. The cell population under study is divided such that one fraction is loaded with a lipophilic cell plasma membrane permeable dye, Calcein UltraGreen™ AM, that is hydrolyzed upon cellular uptake by cytoplasmic esterases to yield Calcein UltraGreen, a highly fluorescent and well-retained and membrane-impermeable molecule.
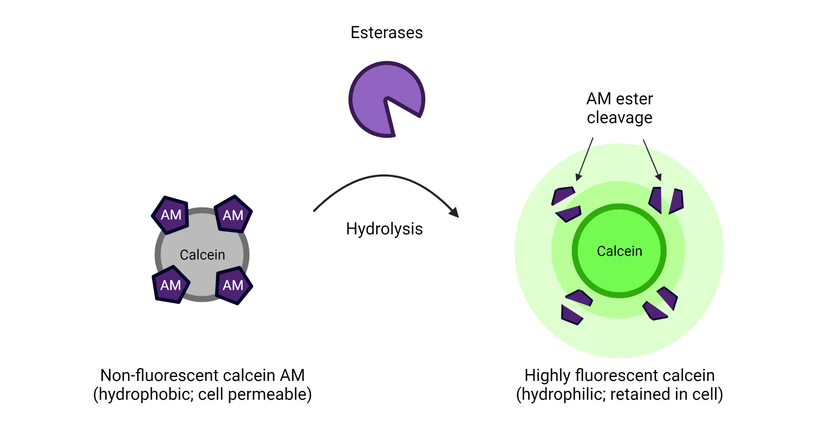
Calcein AM hydrolysis. Non-specific intracellular esterase cleavage of AM ester groups converts calcein AM into highly fluorescent calcein.
The other fraction is loaded DiD, which is a lipophilic membrane dye that diffuses laterally to stain the entire cell membrane in deep red fluorescence upon incorporation into membranes. DiI, DiO, DiD and DiR dyes are a family of lipophilic fluorescent stains for labeling membranes and other hydrophobic structures. The fluorescence of these environment-sensitive dyes is greatly enhanced when incorporated into membranes or bound to lipophilic biomolecules such as proteins although they are weakly fluorescent in water. They have high extinction coefficients, polarity-dependent fluorescence and short excited-state lifetimes. Once applied to cells, these dyes diffuse laterally within the cellular plasma membranes, resulting in even staining of the entire cell at their optimal concentrations. Among them DiD is well excited by the 633 nm He-Ne laser, and has much longer excitation and emission wavelengths than those of DiI, providing a valuable alternative for labeling cells and tissues that have significant intrinsic fluorescence.
Once the fractions have been loaded sufficiently according to the experimental cell type, the two fractions are mixed and incubated under coculture conditions. Calcein UltraGreen is transferred to the DiD-stained cells through gap junctions. The assessment of this uptake can be monitored by fluorescence imaging or flow cytometry.
Sample Protocols
The following example protocols can be used as a guideline and should be optimized according to experimental needs.
Cell staining protocol for plasma membrane dye (Calcein Ultragreen AM)
- Grow cells in cell culture medium in 6-well cell culture plates.
- Remove the cell culture medium and add 0.5 mL of Calcein Ultragreen AM working solution.
- Incubate cells at 37 °C for 10-20 minutes. Note Incubation time should be optimized for each cell line.
- Remove the dye working solution and wash cells with GAP Junction Assay buffer.
Note
For the adherent cells, detach cells from the plate using rubber policeman. - Resuspend cells in cell culture medium
Note
The nonionic detergent Pluronic® F-127 can be used to increase the aqueous solubility of AM esters. Avoid long-term storage of AM esters in the presence of Pluronic® F-127.
Cell labeling protocol for lipophilic membrane dye (DiD)
- Grow cells in cell culture medium in 6-well cell culture plates.
- Remove the cell culture medium and add 0.5 mL of DiD working solution.
- Incubate cells at 37 °C for 10-20 minutes. Note Incubation time should be optimized for each cell line.
- Remove the dye working solution and wash cells with GAP Junction Assay Buffer.
Note
For the adherent cells, detach cells from the plate using rubber policeman. - Resuspend cells in cell culture medium.
GAP junction assay
- Mix Calcein stained cells and DiD labelled cells with 1:1 ratio and plate them in wells.
- For fluorescence microscopy, add 50 µL of each into the well of a 96-well plate and mix well.
- For flow cytometry, add 500 µL of each into the well of a 6-well plate and mix well.
- Incubate cells at 37 °C for 2-3 hours.
- Assess and analyze the cells using either a flow cytometer or fluorescence microscope.
Details of Flow Cytometry Analysis
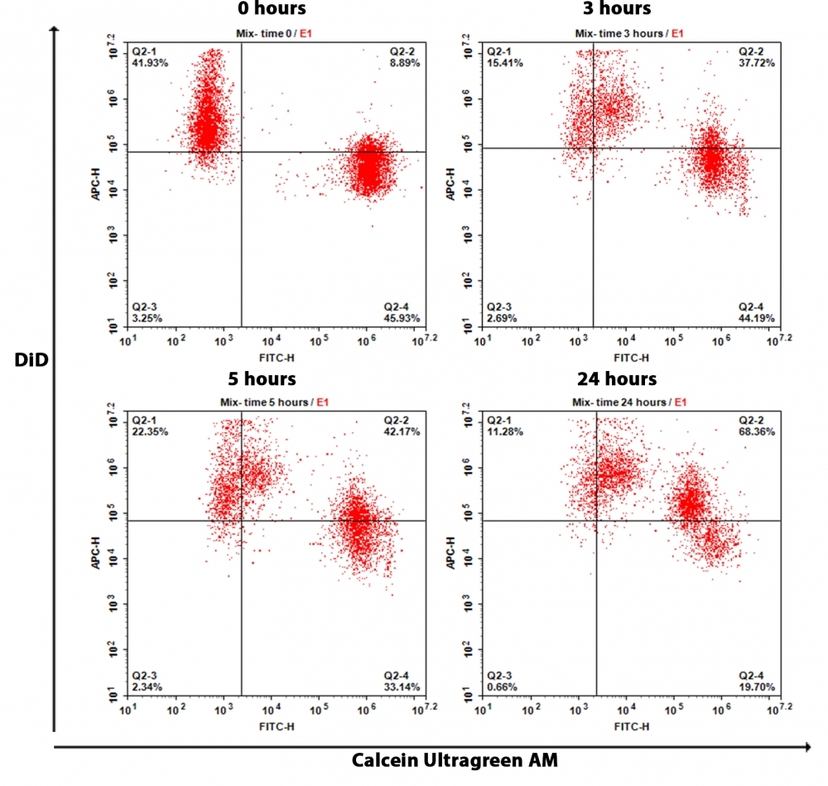
GAP junctions analyzed by flow cytometry. HeLa cells were stained with Calcein Ultragreen AM and DiD separately as per the protocol. Cells were mixed well with 1:1 ratio and replated with cell culture medium. Response was measured using NovoCyte flow cytometer (ACEA Biosciences) with FITC and APC channel. As time progress, Q2-2 population (double positive population) increases.
Note
For the adherent cells, detach cells from the plate using rubber policeman.
For the adherent cells, detach cells from the plate using rubber policeman.
Once cells are in suspension or for cells in suspension, wash cells twice with DPBS or buffer of your choice. Resuspend cells in HHBS or DPBS or buffer of your choice and measure the response with 530/30 nm filter (FITC channel) and 660/20 nm filter (APC channel).
The success of the procedure will dictate how the data should be gated. Clear groupings indicate an ideal experiment, but a lack of clearly separated populations strongly implies one or more issues. As is typical for most cell activity experiments, use negative and positive controls to calibrate and discern meaningful data.
Details of Fluorescence Visualization
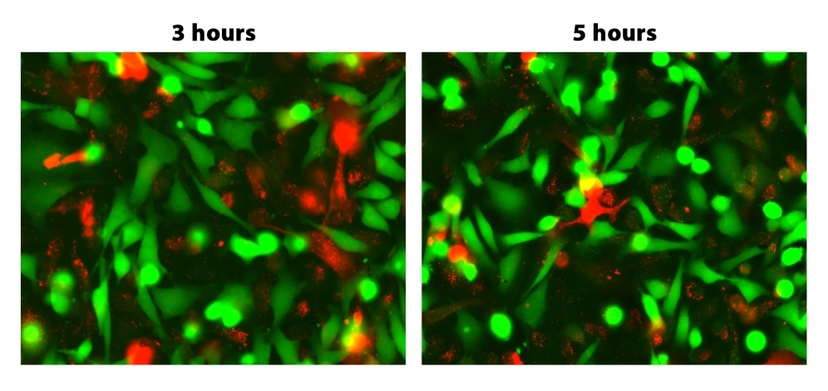
GAP junctions analyzed by fluorescence microscopy. HeLa cells were stained with Calcein Ultragreen AM and DiD separately as per the protocol. Cells were mixed well with 1:1 ratio and replated with cell culture medium. Images were acquired using a fluorescence microscope with the FITC and Cy5 filter sets. As time progresses, double positive population of Calcein Ultragreen AM and DiD increases.
Most gap junction assays, including the one used here to demonstrate, have an incubation timeframe that has been tested and optimized to provide enough time for cells to begin displaying double positive signal (cells that will generate both green and red fluorescence) due to cytoplasmic interchange via gap junctions. Cell populations are likely to continue to increasingly show this double positive trend as time goes on, although cell type and experimental design will affect when this tendency will plateau and/or begin to decrease. The image above shows images at both 3 and 5 hours after initial cocultured incubation, as an example.
Optimization and Troubleshooting Experimental Problems
Based on experimental need, either prepared kits such as demonstrated earlier in this article can be used, or the same principles can be applied on a case-by-case basis. When building a procedure in-house, each aspect must be tested and optimized. For either flow cytometry or fluorescence microscopy platforms, the incubation time and dye concentrations must be assessed for best results, and other platform-specific considerations may come into play as well as variation in behavior based on cell lines.
Gap junction assay incubation time optimization testing in HeLa cells. Cells were washed with DPBS (No Ca+2 and Mg+2), then stained with Calcein AM (5 µM) and DiD (2.5 µM) for 10 minutes at 37C separately. Cells were washed with DPBS twice and mix it together and re plated with complete medium. Cells were collected at the indicated times, washed twice with DPBS, then resuspended in DPBS for flow cytometry procedure. Population of double-positive stained cells in quadrant 2 (Q2) increased as time increased up to the 5 hour mark, then steadied and began to decrease, until a net reduction of the Q2 population was shown at the 24 hour mark.
Although upgraded reagents such as Calcein Ultragreen AM give better performance, cheaper classic options such as Calcein AM are still used frequently. In either case, cellular incubation time should be balanced between functionality and laboratory feasibility. For the image above, testing showed that any incubation longer than 5 hours provided little more data, and in fact less as time went on. This knowledge, despite the relatively low toxicity of the dyes, can inform not only procedure starting times (to permit adequate but not over-incubation) to achieve the best possible experimental results.
Gap junction assay DiD dye optimization. Cells were washed with DPBS (No Ca+2 and Mg+2). HeLa cells were stained with DiD at different concentrations (0.25, 0.5, and 1 µM) for 10 minutes at 37°C. Cells were washed with DPBS twice and re plated with complete medium on a 96- well plate. Images were acquired at indicated times and bright field (BF) and Cy5 images were overlapped. As is evident by the imaging, cells are healthy with both 0.25 and 0.5 µM concentrations, but significantly less so at 1µM.
For determining ideal dye concentrations, the cells should be incubated with a range of molarities. Many kits and protocols will have recommended ranges, which are usually the best starting point. Even for dyes that have minimal cytotoxicity, high concentrations will frequently have deleterious effects to the health and proliferation rates of the cell population.
Note
For the adherent cells, detach cells from the plate using rubber policeman.
For the adherent cells, detach cells from the plate using rubber policeman.
Conclusion
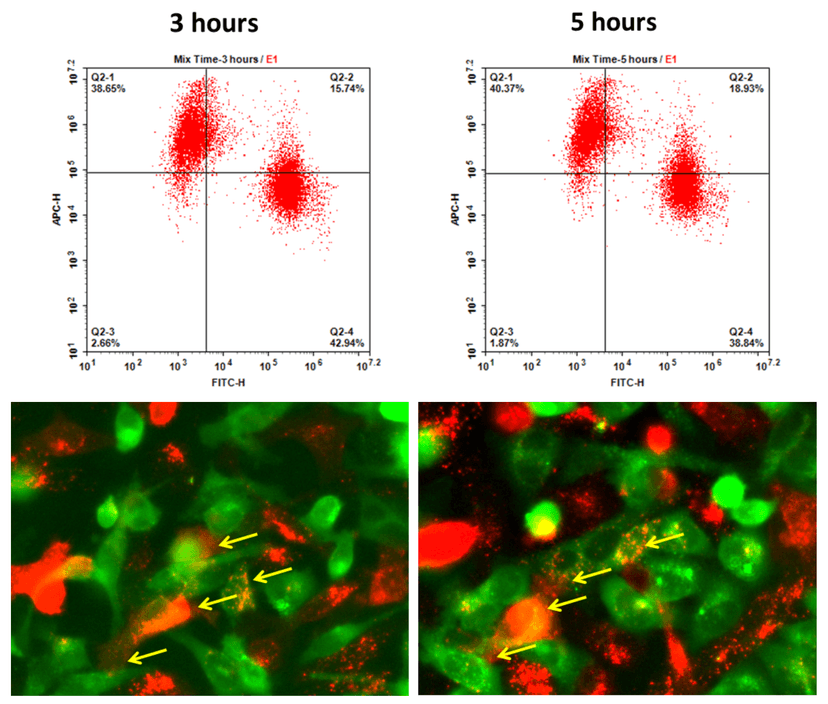
HeLa cells were washed with DPBS (No Ca+2 and Mg+2) and stained with Calcein Ultragreen AM (0.5 µM) and DiD (0.5 µM) for 10 minutes at 37°C separately. Cells were washed with DPBS twice and mix it together and re plated with complete medium. Cells were collected at indicated times, washed twice with DPBS, then resuspended in DPBS and flow cytometry was performed. Yellow arrows indicate double-positive tracked cells. Top row are flow cytometry analyses, and bottom row are fluorescence microscope images, taken at the listed incubation times.
Products
Table 1. Gap Junction Tracing Kit
Cat# ▲ ▼ | Product Name ▲ ▼ | Unit Size ▲ ▼ |
23600 | Cell Meter™ Fluorescence Gap Junction Tracing Kit | 100 Tests |
Table 2. Specifications for calcein AM and calcein AM derivatives.
Product ▲ ▼ | Ex (nm) ▲ ▼ | Em (nm) ▲ ▼ | Spectrum ▲ ▼ | Unit Size ▲ ▼ | Cat No. ▲ ▼ |
Calcein Blue, AM *CAS 168482-84-6* | 354 nm | 441 nm | 1 mg | 22007 | |
Calcein UltraBlue™ | 359 nm | 458 nm | 1 mg | 21908 | |
CytoCalcein™ Violet 450 *Excited at 405 nm* | 406 nm | 445 nm | 1 mg | 22012 | |
CytoCalcein™ Violet 500 *Excited at 405 nm* | 420 nm | 505 nm | 1 mg | 22013 | |
Calcein, AM *CAS 148504-34-1* | 501 nm | 521 nm | 1 mg | 22002 | |
Calcein, AM *UltraPure grade* *CAS 148504-34-1* | 501 nm | 521 nm | 1 mg | 22003 | |
Calcein, AM *UltraPure grade* *CAS 148504-34-1* | 501 nm | 521 nm | 20x50 µg | 22004 | |
Calcein Orange™ diacetate | 531 nm | 545 nm | 1 mg | 22009 | |
Calcein Red™ AM | 562 nm | 576 nm | 1 mg | 21900 | |
Calcein Deep Red™ AM ester | 643 nm | 663 nm | 1 mg | 22011 |
Table 3. Lipophilic tracers for labeling cell membranes in live and fixed cells and tissues.
Product Name ▲ ▼ | Ex/Em (nm) ▲ ▼ | Filter Set ▲ ▼ | Unit Size ▲ ▼ | Cat No. ▲ ▼ |
DiOC2(3) iodide [3,3-Diethyloxacarbocyanine iodide] | 482/500 | FITC | 25 mg | 22038 |
DiOC3(3) iodide [3,3-Dipropyloxacarbocyanine iodide] | 482/500 | FITC | 25 mg | 22039 |
DiOC7(3) iodide [3,3-Diheptyloxacarbocyanine iodide] | 482/500 | FITC | 25 mg | 22040 |
DiOC16(3) perchlorate [3,3-Dihexadecyloxacarbocyanine perchlorate] | 482/500 | FITC | 25 mg | 22042 |
DiOC5(3) iodide [3,3-Dipentyloxacarbocyanine iodide] | 482/500 | FITC | 25 mg | 22045 |
DiOC6(3) iodide [3,3-Dihexyloxacarbocyanine iodide] | 482/500 | FITC | 25 mg | 22046 |
DiO perchlorate [3,3-Dioctadecyloxacarbocyanine perchlorate] | 482/500 | FITC | 25 mg | 22066 |
CytoTrace™ CM-DiI | 548/563 | TRITC | 10x50 µg | 22057 |
DiIC12(3) perchlorate [1,1-Didodecyl-3,3,3,3-tetramethylindocarbocyanine perchlorate] | 549/563 | TRITC | 25 mg | 22035 |
DiIC16(3) perchlorate [1,1-Dihexadecyl-3,3,3,3-tetramethylindocarbocyanine perchlorate] | 549/563 | TRITC | 25 mg | 22044 |
Resources
Application Note:
Digital Catalog Pages:
Selection Guide:
Webinar:
References
Neuroinflammation Leads to Region-Dependent Alterations in Astrocyte Gap Junction Communication and Hemichannel Activity
Tight junctions: from simple barriers to multifunctional molecular gates
Adherens and tight junctions: Structure, function and connections to the actin cytoskeleton
Gap junction and hemichannel-independent actions of connexins on cell and tissue functions-An update
Properties of gap junction blockers and their behavioural, cognitive and electrophysiological effects: Animal and human studies
Gap Junction Coupling and Calcium Waves in the Pancreatic Islet
Gap junction plasticity as a mechanism to regulate network-wide oscillations
The roles of connexins and gap junctions in the progression of cancer